The Future of Animal Feed: Animal by-products and insects
This section looks further at animal by-products and insects
Animal-based protein sources have been used primarily to counteract some of the drawbacks of plant-based feeds such as their relatively low protein content and presence of several anti-nutritional factors that reduce nutrient availability and tackle major sustainability issues such as land use and global warming potential [Lasekan, Bakar & Hashim, 2013; DiGiacomo & Leury, 2019]. While processed animal proteins (PAPs) of fish origin (i.e., fish meals) have been a common feed ingredient for many livestock species, in many countries legislation prohibits the use of PAPs from other species (e.g., avian PAPs) to prevent potential disease outbreaks like the epidemic of bovine / transmissible spongiform encephalopathies (BSE/TSE) in the 1980s [Lecrenier et al., 2020; Woodgate & Wilkinson, 2021]. Advancements in PAP rendering technologies and the controlling and regulatory processes may lead to lifting of such bans, as happened recently in the European Union (EU) [EU Commission, 2021]. The EU now allows for swine PAPs to be used in avian diets and vice versa, while insect PAPs can be used in either. However, ruminant PAPs are still prohibited to prevent a reoccurrence of TSE outbreaks, with the exception of milk, collagen and gelatine that can be used only for non-ruminants [Ricci et al., 2018].
Insects are also becoming increasingly popular both as potential feeds and human foods, with insect meals typically containing between 50 – 82% crude protein as well as other important nutrients (e.g., calcium, iron) [Madau et al., 2020]. As natural decomposers, insects can be reared in a great variety of substrates from feedstock material to waste, therefore converting unwanted substrates into sources of high-quality protein and healthy fats. To date, insect protein meals, mainly from five species have been explored and commercially exploited in bird and pet feeds, while their introduction in the livestock feed sector is a subject of further research [Manceron et al., 2014; Kim et al., 2019; van Huis et al., 2021].
Environmental implications
Land degradation, land use change and land availability related impacts
As a circular approach, using swine, poultry and ruminant PAPs in livestock feeds can help achieve similar overall environmental benefits to those discussed for protein obtained from food waste and industry waste streams, and cellular protein reared on waste. The production of insect protein for livestock feed at commercial scales however, presents slightly more complex interactions with the environment [Madau et al., 2020; van Huis et al., 2021].
Insect farming can potentially reduce land use requirements for protein production e.g., up to 98% when compared to soybean-fishmeal protein mixtures [van Huis & Oonincx, 2017]. However, it should be noted that insect farms (i.e., facilities) are not landless units and therefore unintended land-use impacts may become relevant for insect farming too as the sector expands i.e., with the displacement of other agricultural activities or driving land-use change in urban areas [Doi & Mulia, 2021].
Greenhouse gas emissions, atmospheric pollution and fossil fuel depletion
Mass rearing of insects for livestock feeds overall generates less GHG emissions when compared to conventional protein sources (e.g., fish meals, soy). However, it requires higher amounts of energy mainly for indoor climate regulation, an important condition for optimal insect growth as they are poikilotherms [van Huis & Oonincx, 2017; van Zanten et al., 2015]. Despite the high energy requirements, insect farming is not heavily dependent on fossil fuel because its typical farming operations involve the use of electricity. Therefore, there is a potential to fulfil these requirements through renewable energy sourcing and consequently further reduce the carbon footprint of livestock feed overall [Wang & Shelomi, 2017]. It is important to consider that insect farming is still at its infancy and there is a lot of room for improvement regarding resource efficiency, particularly considering the ability of insects to be reared on a wide range of substrates including various wastes [Bosch et al., 2019].
Nitrogen and phosphorus related impacts
In addition, rearing insects on manure produced by livestock (i.e., circular approach) has the potential to further reduce total nitrogen concentrations in agricultural soils by up to 62% [Elahi et al., 2022]. Further research should explore synergies and potential benefits from using different industry waste streams (e.g., food wastes) to identify optimal insect rearing strategies depending on specific regional agri-environmental policies; for example, organic wastes from livestock and households could be funnelled towards insect farming to help reduce overall concentrations of nitrogen and phosphorus in designated vulnerable zones (i.e., Nitrogen Vulnerable Zones, Phosphorus Vulnerable Zones) mitigating acidification and eutrophication impacts [Huygens et al., 2020].
Impacts to water quality and depletion of water resources
Although insects rely heavily on water for their growth, the overall water requirements for mass insect rearing are still significantly lower compared to conventional protein crop production [Wang & Shelomi, 2017]. The benefits of insect farming on depletion of water resources and water quality can be even greater if wastewater sludge and slurries are considered as substrates for rearing (i.e., upcycling of wastewaters to valuable protein). Under such scenarios, it is important to note that alternative uses of wastewater and their benefits can vary across geographies. For example, wastewater could be used for the production of energy, irrigation in conventional agriculture, or domestic (e.g., showering, washing) and non-domestic use (e.g., firefighting, swimming pools), all of which could be prioritised differently depending on countries’ water-stress levels [Zhao et al., 2019; Malone & Newton, 2020].
Impacts to biodiversity
As discussed for the cellular and circular agriculture alternatives above, adopting soilless protein production strategies such as protein from animal and insect PAPs can have several indirect positive implications for the conservation of global biodiversity, primarily through mitigating habitat loss, fragmentation and degradation impacts caused by conventional crop production [Jansson & Berggren, 2015]. However, it is important to consider that poorly managed mass rearing facilities may introduce risks for displacement of local species, i.e., through uncontrolled mixing of farmed and wild insect populations [Jansson & Berggren, 2015; van Huis et al., 2021].
Economic implications
Production and supply economics and resilience to extreme events
The economic feasibility of mass rearing of insects has been a topic of debate and conflict in recent literature. Due to high requirements for energy, the relatively low prices of competing conventional protein sources, and challenges in the marketability of insects as feed for livestock systems in Europe and Western countries, insect farming has not yet been exploited extensively at industrial scales [Arru et al., 2019]. On the other hand, insect farming can help avoid costs associated with synthetic and chemical inputs (i.e., does not require fertilisers), fossil fuel use (i.e., relies more on renewable energy), transportation (i.e., insect farm facilities can be located in or near urban areas) and labour [DiGiacomo & Leury, 2019; WWF, 2021]. Considering also the potential for insects to be reared on industry wastes (i.e., circular business model) further increases its potential cost-effectiveness through discounts in waste disposal of associated industries [Chia et al., 2019; Madau et al., 2020]. This diversity of potential synergies with other industries suggests that insect farming can be a resilient protein production system, robust to changes in availability and pricing of rearing substrates / inputs. Furthermore, its resilience can be enhanced with developments in the renewable energy sector that may enable uninterrupted and less expensive supply of electricity from renewable sources [DiGiacomo & Leury, 2019; WWF, 2021; van Huis et al., 2021].
Social implications
Nutritional value and animal growth
Insects are natural decomposers and great at converting energy embedded in organic matter into high concentrations of edible protein ranging between 35% and 82% [DiGiacomo & Leury, 2019; WWF, 2021]. In addition to this, insect meals provide livestock with important bioactive compounds and nutrients, such as iron, zinc, antimicrobial peptides, chitin, and lauric acid that can improve gut health and promote growth [Gasco et al., 2018; Madau et al., 2020]. Although evidence indicates that insects are a great source of high-quality protein for livestock, it is important to consider that their nutritional value may vary between insect species and depending on the substrate they are reared on [Oonincx & Finke, 2021; Pinotti & Ottoboni, 2021; WWF, 2021].
Animal health and welfare
While swine, poultry, and ruminant PAPs are a good source of protein and beneficial nutrients that promote animal growth and gut health, the way they are incorporated to livestock feeds should be carefully considered. Cross-feeding strategies appear to be critical in order to minimise the risks for viral contaminations that can cause significant impairments to animal health and welfare, or for severe diseases caused by prions such as BSE/TSEs.
As discussed in previous Chapters (i.e., protein production from cellular agriculture and food waste), using waste substrates for mass insect rearing may introduce important threats to their health and welfare, such as the accumulation of heavy metals, pesticides, microplastics and other contaminants that can reduce insect growth and increasing mortality rates [Schrögel & Wätjen, 2019]. An important issue to consider in relation to this is the ambiguity around the classification of farmed insects as feed or livestock. The latter could require strict standards to be followed in the insect farming industry to ensure insect health and welfare. Further, insects carrying biological and chemical contaminants are then fed to farmed fish/shellfish or livestock, and the contaminants reach their tissues where they cause various complications such as intestinal blockages, reducing animal appetite and growth.
Social development
Aside from promoting human wellbeing with the upcycling of animal by-products and wastes used for insect rearing, such alternatives present great opportunities for socio-economic growth. Due to the range of insect species that are suitable for consumption by animals, there can be economic, stable, locally produced insect farming solutions that allow smallholder livestock producers globally to be self-sufficient and avoid large costs associated with purchasing of conventional protein feed ingredients (Fig. 6) [Chia et al., 2019]. Furthermore, because insect farming is just starting to develop and considering its requirements for energy, hygienic treatment of waste substrates, and detection of microscopic contaminants, it may be a great driver for innovations in biotechnologies, regulatory and safety assessment systems and policies of the livestock sector, and the food-energy-water nexus overall [Ojha et al., 2021; Sindermann et al., 2021].
Figure 6: Contribution of insect farming to global Sustainable Development Goals from a socio-economic perspective. Source: Chia et al. (2019)
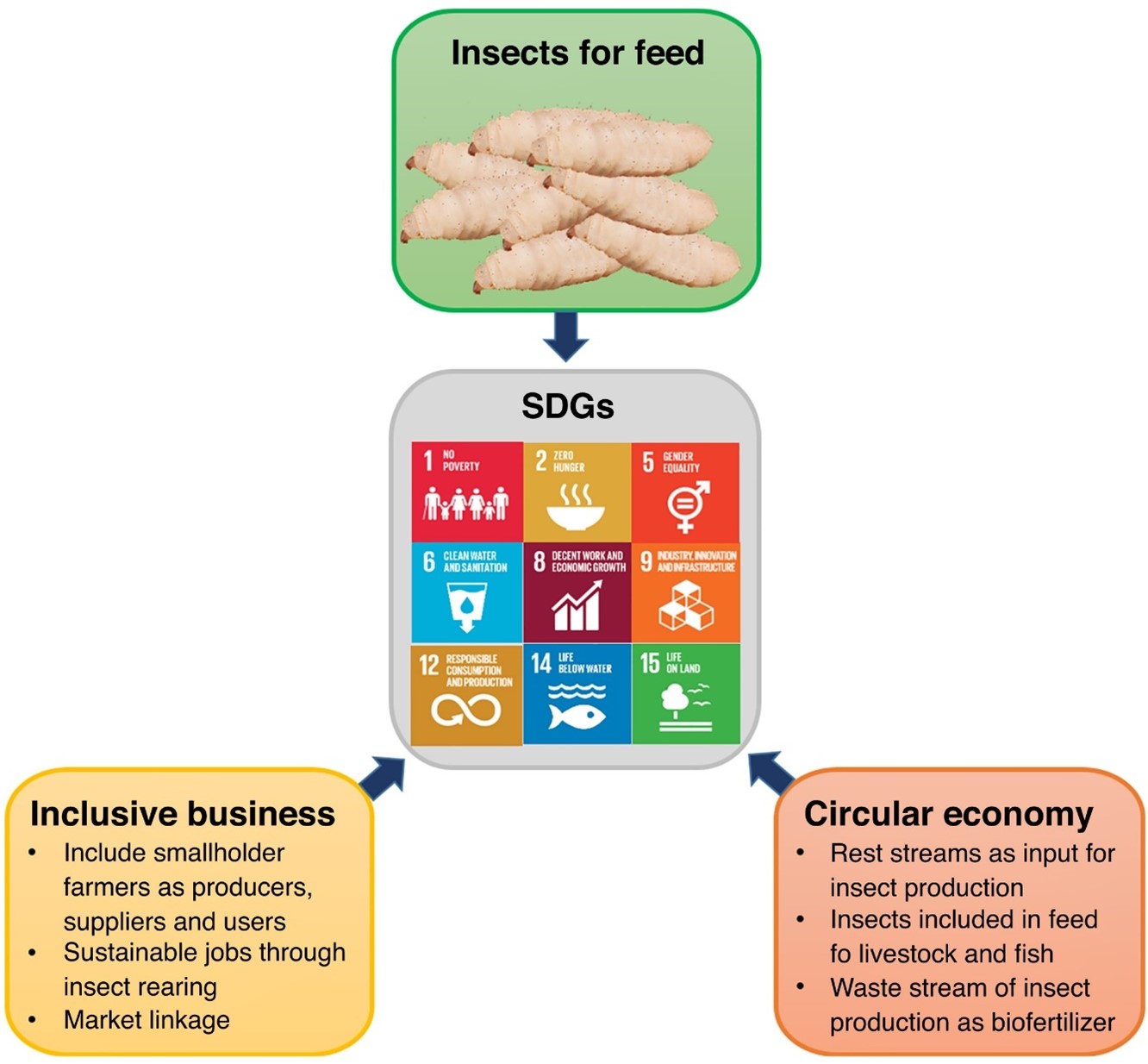
Consumer perception and acceptance
Along with legislation, consumer acceptance and attitudes towards the use of protein feeds from insects and animal by-products present the most important barriers to scale-up of these alternatives. There are major concerns regarding the extent to which religious and cultural factors would affect consumers acceptance of livestock reared on animal and insect PAPs, which could significantly limit the available markets. Such concerns mainly relate to the use of swine or beef PAPs that are considered forbidden in certain religions and cultures. Biases (e.g., disgust) developed through lack of understanding and socio-cultural misinformation significantly contribute to stakeholder distrust towards the safe and successful adoption of alternative proteins in livestock feeds. However, there are still gaps in research and more efforts are required to understand whether circular agriculture alternatives and insects are more acceptable when used as feeds rather than foods [van Huis et al., 2021]. In many cultures, including the Western world, insects reared for feed are viewed as livestock and therefore, consumers require insect rearing systems to adhere to high animal welfare standards. Retailer requirements and livestock certification standards could put further pressures on the growth and adoption rates of such protein feeds, requesting safety assurances to prevent food safety and mislabelling issues [van Huis et al., 2021; WWF, 2021].
Food safety
The re-introduction of PAPs from swine, poultry, and ruminants to livestock feeds raises some critical biosecurity concerns, mainly regarding past experiences of human disease outbreaks like the BSE/TSE epidemic of the 1980s [Woodgate & Wilkinson, 2021]. To minimise such risks, animal by-products and PAPs should be fed strictly cross-species (e.g., swine PAPs to poultry and vice-versa). Livestock feed producers that use PAPs from more than one species should carefully consider potential risks of cross-contamination and avoid mixing and milling such feeds using the same facilities [Lecrenier et al., 2020; Woodgate & Wilkinson, 2021].
Another major food safety risk arises with the mass rearing of insects, which can also be vectors of diseases and lead to pathogen contaminations threatening animal and eventually human health [van Huis et al., 2021]. When reared on livestock manures insects may carry veterinary drug residues, hormones and biological contaminants (e.g., bacteria with transgenic DNA, mycotoxins). When reared on plant and crop production waste, they may be exposed to pesticides and herbicides and mycotoxins. In addition, there is a risk for heavy metals and micro and nanoplastics to be accumulated into insects through waste substrates [Truzzi et al., 2020]. Bioaccumulation pathways then such as “animal feed è livestock è manure è farmed insects è livestock è humans”pose serious threats to human health and overall food safety. In addition to causing impairments in animal performance and welfare as discussed above, contaminants can enter the human food chain through livestock product consumption and cause severe effects like inflammatory responses, disruption on gut microbiota and effects on nutrient absorption, and even chronic inflammations that increase the risks for cancer [Smith et al., 2018; Prata et al., 2020]. The extent of bioaccumulation varies across the different farmed insect species, the growth phase they are in when exposed to contaminants, and the type of biological contaminant, metal or plastic particle [van der Fels‐Klerx et al., 2018; Meyer et al., 2021]. Thus far, studies have shown that the concentrations of both biological and chemical contaminants significantly decrease as insects reach adulthood [BfR, 2019; Schrögel & Wätjen, 2019]. However, more research is required to understand the specific pathways for the different contaminants and quantify bioaccumulation at each key step of these pathways up until human consumption (e.g., insect growth phases, animals digestive tract, livestock meat, milk, eggs) to better inform prevention strategies and regulatory systems [van der Fels‐Klerx et al., 2018]. To date, several mitigation strategies have been established to address the risk of biological contaminants and specifically the removal of pathogens at PAPs production phase. The “kill-step” is a well-used strategy in food safety that involves killing pathogens using thermal processing over time. Common thermal processing practices include pasteurisation, freezing, and heating / cooking. While these strategies are highly effective, there is a need to enhance our understanding of bioaccumulation pathways and processes that can potential change the physicochemical properties of both biological and chemical contaminants to further reduce the risks for food hazards.
Finally, allergens introduced to livestock and humans from insects may pose a serious risk for food safety. Studies have investigated the potential for cross-reactivity between edible insects and “domestic” insects and found that tropomyosin is such a cross-reactive allergen. They further investigated potential treatment methods to reduce allergenicity of edible insects and concluded that the reactivity of immunoglobulin is resistant to thermal processes and enzymic digestion. Therefore, evidence suggests that individuals allergic to “domestic” insects and those who are involved in insect farming should be considered as high-risk groups regarding their exposure to livestock products reared on insects or edible insects [Ribeiro et al., 2021].
Regulatory implications
Regulatory and safety assessment systems should employ state-of-the-art biotechnologies and practices to detect PAPs (e.g., specific immunoassay and polymerase chain reaction methods, infrared microscopy) and frequently reassess the prevalence of classical and atypical forms of BSE/TSEs [BIOHAZ, 2011].
To ensure safe mass rearing of insects for livestock feeds, thorough safety assessments and close monitoring of farming practices should be established, while pathogen resistant genotypes should be explored by future research (e.g., understanding of virome in commercially reared crickets) [de Miranda et al., 2021].
Table 2: Could insects be a sustainable protein source for livestock? The table summarises the main environmental, economic, and social impacts associated with the commercial implementation of insect meals as protein source for livestock feeds.
Environmental Implications
- Insect farming presents opportunities to combat critical environmental pressures. It does not rely on extensive land areas and fossil fuel inputs to the same extent as conventional protein crop production.
- It can be implemented in a wide range of climate conditions and locations, help reduce pressures on land and soil quality, and keep climate-related impacts to a minimum through the use of renewable resources.
- At larger scales it becomes a very energy demanding operation and so it should rely mostly on renewable energy rather than fossil fuel.
- Preliminary evidence points towards significantly lower impacts than conventional protein crop production for biodiversity, water quality, acidification, and eutrophication.
Economic Implications
- High start-up and operational costs make the exploitation of insect farming at industrial scales difficult.
- The lack of government financial support, private investments, and the competition for resources with subsidised industries create suboptimal conditions for growth of this production system, particularly in the absence of a stable market.
- Standardisation and assurance of production standards and inclusion limits may be required to encourage retailers to support livestock producers to use insect meals.
Social implications
- Insects can be reared on waste substrates, therefore upgrading them to healthy fats and proteins.
- Adding value to waste promotes a strong sense of caring for the environment.
- Their production relies less on manual labour than conventional protein sources and presents opportunities to promote innovation through automation of farming operations.
- There is a threat that insects can be disease vectors particularly if reared on waste, and that using insect processed proteins may lead to disease outbreaks.
- Strict legislation on waste substrates, insect processing, and feeding strategies are needed to minimise feed and food safety risks from insects as vectors of diseases.
- Further understanding agri-food stakeholders’ perspectives on insect farming is imperative to combating biases, misinformation, and to work towards silver bullet solutions.
Tables 3-6 below summarise the potential opportunities and risks to sustainability and food safety associated with the commercial implementation of the alternative protein ingredients discussed in this REA.
Table 3: Environmental opportunities and risks associated with the incorporation of alternative protein sources in livestock feeds
Environmental Implications | Opportunities | Risks |
Land degradation, land use and land availability related impacts |
Diversify and shift protein production from the global South reducing deforestation and land-use change pressures in endangered ecosystems (e.g., Amazon) – e.g., insect farming uses 90% less land compared to soy production. Soilless crop growing methods resulting to more land available for food production and healthier soils overall.
|
Poor management of former arable areas leading to abandoned and deserted lands. Geographical shift in protein production increasing feed-food competition for land in the global North and knock-on effects of land-use.
|
Greenhouse gas emissions, atmospheric pollution and fossil fuel depletion |
Reduced events of biomass burning and deforestation. More land occupied by trees leading to healthier atmosphere. Less synthetic and chemical inputs required for soilless and circular alternatives, therefore less energy required and emissions for their production. |
Specific operations of soilless alternatives (e.g., drying process at seaweed farming) may require large amounts of energy, which can increase fossil fuel depletion pressures unless renewably sourced. |
Impacts to biodiversity |
Reduced synthetic and chemical inputs improving conditions for terrestrial & aquatic life. Reduced events of biomass burning and deforestation leading healthier and less fragmented habitats and ecosystems. Seaweed farming creating new habitats for aquatic wildlife.
|
Invasiveness/weediness of GM/GE crops. Gene flow from GM/GEs threatening wild genotypes. Poor management of mass insect rearing facilities resulting to contamination of ecosystems with non-native species.
|
Nitrogen and phosphorus related impacts |
Less synthetic fertilisers for crop production leading to reduced leaching to soils and water, therefore reducing acidification & eutrophication pressures. Recycling of N and P from food waste, former foods, animal by-products, and industry by-products through circular agriculture, reducing nutrient leaching at waste disposal.
|
Freshwater algae and seaweed farming leading to increased N and P concentrations in aquatic ecosystems. Changes in manure composition of livestock due to consumption of alternatives, resulting to increased N and P deposition at field application or emissions at storage.
|
Impacts to water quality and depletion of water resources
|
Reduced requirements for irrigation due to more resilient GM/GE genotypes and soilless alternatives. Less synthetic and chemical inputs leading to healthier groundwater for human consumption. Upcycling of wastewater through circular and cellular agriculture. |
Increased water requirements for hydroponics, insect farming, and food waste processing at commercial scales. |
Table 4: Economic opportunities and risks associated with the incorporation of alternative protein sources in livestock feeds.
Economic Impacts | Opportunities | Risks |
Production and supply economics |
Reduced costs associated with synthetic and chemical inputs (e.g., fertilisers, pesticides, herbicides). Reduced costs for transportation and import of protein feeds from long distances / international, as well as easier access to labour with cellular and circular agriculture hubs or insect farms located closer to feed manufacturers and livestock production systems.
|
High capital / start-up costs for implementation of some soilless alternatives (e.g., seaweed, insect farming) at commercial scales. Reduced availability of novel technologies required for commercialisation. High operating costs due to energy requirements, especially as long as renewable energy prices remain high. High operating costs due to energy requirements, especially as long as renewable energy prices remain high.
|
Robustness to economic uncertainties and extreme events |
Protein production from GM/GE and local crops being more resilient to extreme climate and weather events and interruptions in supply chains (e.g., reduced losses due to long delays in transportation). Uninterrupted and robust to global trading dynamics supply by diversifying with soilless alternatives and shifting to increased local protein production. |
Sensitive to volatile energy prices due to high energy requirements. Cost-effective commercial implementation depending largely on advancements in biotechnology. |
Table 5: Social opportunities and risks associated with the incorporation of alternative protein sources in livestock feeds.
Social Implications | Opportunities | Risks |
Animal health and welfare |
Crude protein content as high as 93% (e.g., poultry PAPs) Good sources of fats and bioactive compounds that promote animal gut health and growth Animal by-products, insects, and food wastes characterised by enhanced bioavailability of nutrients compared to most plant-based protein sources Reduced risk for animal diseases (e.g., Foot and Mouth disease) caused by poor transportation and storage conditions of protein crops
|
Uncertainties around complete GM genome and functionality Acceptability by livestock of alternative and novel feeds resulting to inefficient feeding and impaired growth Poor hygienic processing in circular streams (e.g., food waste) resulting to accumulation of biological (e.g., mycotoxins) and chemical (e.g., nano-plastics) contaminants in livestock tissues
|
Social development |
Increased feed availability due to shorter supply chains Reduced heavy-duty on-farm labour Innovation in production / supply chains
|
Automation of production and supply chains for alternative proteins resulting to higher unemployment rates in agriculture Reduced protein feed production from the global South resulting to impoverishment of rural communities
|
Consumer perception and acceptance | Sustainable alternatives promote a “feel good” factor |
Lack of understanding and misinformation Increased incidents of feed fraud and mislabelling to make livestock products more appealing to consumers Some alternatives like insects or food waste may promote a “disgust” factor |
Table 6: Food safety related opportunities and risks associated with the incorporation of alternative protein sources in livestock feeds
Food safety | Opportunities | Risks |
Biological contamination | Less mycotoxin outbreaks caused by storage and transportation implications. |
Uncertainties around complete GM genome and functionality. Increased risk for viral disease outbreaks from animal by-products, such as BSE/TSEs. Poor processing of wastes for protein feed production resulting in increased risk for pathogens to reach humans.
|
Chemical contamination | Less pesticides / uptake of heavy metals from irrigation waters. | Poor processing of wastes for protein feed production resulting in increased risk for contamination of humans by microplastics, nano-plastics, and packaging remnants through bioaccumulation. |
Allergenicity | GM/GE crop variants potentially reducing the expression of proteins causing allergies. | Major allergens introduced in human foods through bioaccumulation from several alternative feeds such as GM/GE crops, legumes, seaweed, and food waste. |