Technology trends in 3D printing of food
Within the technologies currently used for 3D printing of food a number of engineering research and development trends can be observed that mostly aim to optimise certain issues around printing specific foodstuffs.
Within the technologies currently used for 3D printing of food a number of engineering research and development trends can be observed that mostly aim to optimise certain issues around printing specific foodstuffs. Only after significant technological progress is achieved in these areas will sub-application areas of 3DFP be able to gain commercial viability and enable growth of certain market segments.
3.1 Optimising hardware and processes for different food ingredients
As research into the challenges of 3DFP has increased rapidly over the past five years it has become clear that on the whole 3DFP is still not a robust process for most mixtures of food ingredients and continuous failsafe printing over longer periods of time with consistent high quality results is rarely achievable. Moreover, the size of printable objects is currently limited to a few centimetres in dimension, and with increasing size the complexity of shapes has to reduce considerably. Hence, most novel and experimental food inks are usually printed as simple shapes. The scientific study of the physical and chemical properties of various food ingredients has also shown over many decades that most food ingredients, and in particular mixtures, show a high degree of complexity with regards to chemical, physical, electrostatic and molecular crosslinking properties, which makes exact scientific assessment and prediction of their rheology and printability difficult. Hence, in particular in extrusion–based printing, engineers rely on semi-empirical relations in order to model and predict rheological properties of food ingredients (Wilms et al., 2021). The focus of engineering improvements is mostly on two aspects of the printing process:
- Product flow during printing, known as extrudability, which is defined by nozzle geometry and extrusion force.
- Product stability after extrusion, known as buildability, which concerns the rheological properties of the material after printing and its ability to resist deformation when the next layer is deposited.
In order to streamline approaches for improving printability of food ingredients from a hardware and an ingredient formulation engineering perspective an engineering “tool box” approach has been suggested recently that provides a practical starting point for qualitative and numerical analysis and modelling methods that can be applied to optimise printer parts and parameters for different ingredients. This may help the 3DFP field to consolidate empirical approaches and speed up technical developments in the future (Wilms et al., 2021). Besides improving printability, the issue of up-scaling the technology is currently addressed mostly by developing multi-nozzle systems, or by adding more printers in jointly controlled arrangements, such as ‘printer farms’.
Also improvements of printer control through software innovation are expected through the standardisation of applications such as at-line, computer vision based flow rate tracking and developments such as using AI approaches for online adjusting of printer settings during the print run, helping to make 3DFP a more robust technology (Ma et al., 2023). A more detailed presentation of hardware and software engineering approaches is beyond the scope of this report.
3.2 Integrated mixing and cooking functions for extrusion printing
The idea to integrate ingredient mixing devices with shaping and cooking appliances was publicised over ten years ago propagating the concept of ‘digital gastronomy’ that got some media attention at the time, proposing designs for kitchen appliances such as the ‘virtuoso mixer’, the ‘digital fabricator’ and the ‘robotic chef’. However, despite considerable progress in the robotics field over the intervening years, these concepts have not made much progress in delivering commercially viable appliances to the market (Zoran & Coelho, 2011).
Exact proportions of ingredients are not only essential for the success of the 3D printing process, but are also the conceptual foundation of suggested application areas, such as personalisation of nutrients in 3D printed food items. However, in most of the current extrusion based systems, mixing is performed as a pre-processing step independent from the printing system. Liquid/liquid mixing systems, such as the Dicov3ry system made by Structur3D Printing (Canada) have been reported and tested as potentially suitable for integration with food printers, for example to 3D-print fruit-based ingredients, but Integrated mixing systems are currently mostly at the working-prototype stage in research laboratories (Tan et al., 2018; Tomašević et al., 2021). Diañez and coworkers have tested successfully a liquid/liquid mixing system of their own design for the printing of oleogels (Isabel Diañez et al., 2022). Despite the fact that liquid/solid mixing is one of the most prevalent processes in cooking and the food industry, well working solid/liquid mixing systems integrated with 3D food printers are still not widely implemented outside of research laboratories, despite their frequent use in pharmaceutical applications. They would open up possibly the largest range of new applications around customisation and personalisation as well as controlled food additives addition, however only a few systems are described or tested with a real-world product. One example was recently published by Diañez and coworkers for the printing of foods for dysphagia patients and shows that innovation in this area is achievable using simple mechanical engineering solutions, however it is acknowledged that integrated ingredient mixing requires still further R&D to deliver standardized products (I. Diañez et al., 2021).
Like with ingredient mixing, integrated cooking during printing instead of separate post-processing of the printed product appears appealing, however, due to the fact that the change in physical and chemical properties of the cooked state mostly interferes with layer building, robust convincing concepts are at present rare. Moreover, one of the main challenges is the fact that controlling heat at a precision that would be required for quasi-simultaneous cooking while printing is very challenging even when using lasers or ohmic heating concepts (Khodeir et al., 2021). Cooking of 3D printed chicken meat paste with blue (for cooking the core) and infrared lasers (for browning the surface) has been tested in the CreativeMachines lab of Prof. Hod Lipson at Columbia University, and Israeli startup SavorEat with its Robot Chef food printer for plant-based meat products that cooks plant proteins during the extrusion process, are to date the most advanced examples of integrated cooking processes with claims to build commercially viable appliances in the future (Everett, 2021).
3.3. 4D food printing
4D printing is a technology that uses a 3D printer to print structures with materials that can change over time (hence 4D), or upon exposure to a physical or a chemical stimulus some time after printing. Material change behaviours in 3D printing were initially mostly explored with regards to shape changes using mainly four principles, self-assembly of elements, deformation mismatch, bi-stability, and shape-memory effects, and a number of technical applications for printed, shape changing structures have been found in areas from biomedicine to construction (Yang et al., 2020; Z. Zhang et al., 2019). 4D printing of food has been explored over the past five years applying the same physical and chemical principles using food ingredients. Apart from changing shape, for example after baking or drying, reproducible change of texture, colour and flavour have been explored with a number of food ingredients (Ghazal et al., 2019, 2021, 2022). To elicit 4D effects, external stimuli, such as contact with water/moisture, heat, light or change of pH etc. need to interact with 3D printed composite mixtures of food materials. Possibly the first 4D printed edible item was a film of a mixture of starch, cellulose, agar and proteins produced at MIT in 2017 that changed shape after contact with water (Wang et al., 2017). Also shape change after drying of 2D printed structures onto edible films has been explored repeatedly, for example by exposing pumpkin paste with different salt concentrations to different temperatures to achieve certain shapes from a flat printed product (fig 7).
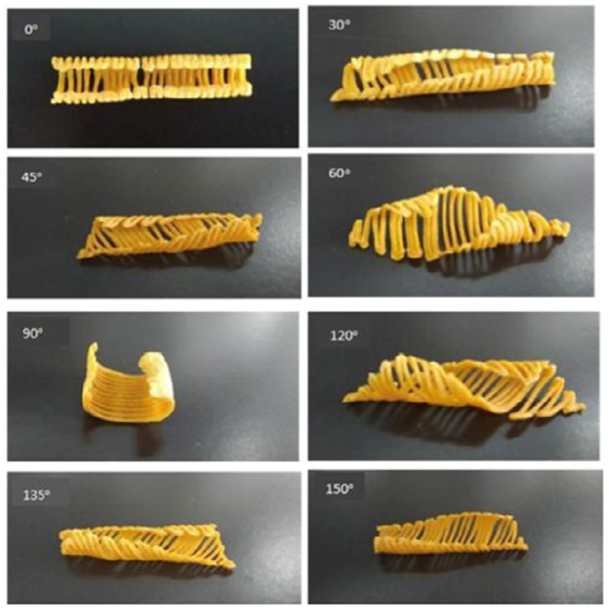
Figure 7: Example of 4D shape change after drying of a flat structure made of pumpkin paste printed onto an edible film. Source: F. Chen et al., 2021
It is usually the combination of ingredients that enables certain change effects, and a limited number of studies added natural colour changing molecules, or aromatic molecules that change flavour upon certain stimuli to a printable base material. Colour and aroma change has for example been explored in a mixture of mashed potato and purple sweet potato puree, lotus root powder gel, and anthocyanin-potato starch gel, after microwave heating and change of pH (Chen et al., 2021; C. He et al., 2020), and in buckwheat/yellow flesh peach dough in combination with edible gelatine gum-arabic-oil complex coacervates microcapsules, containing (E)-cinnamaldehyde as the (cinnamon) aroma. After microwave heating a two-fold increase of cinnamon aroma was reported (Guo et al., 2021). Ethyl cellulose has recently been tested as an additive for 4D printing applications due to its potential as a shape and colour change enabling ingredient as well as a number of natural ingredients that change flavour or colour upon change in pH (Navaf et al., 2022; Pulatsu et al., 2022).
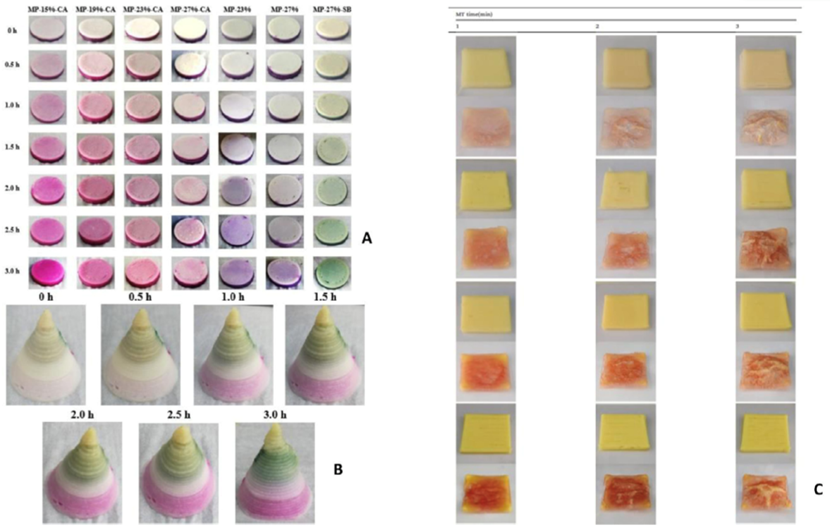
Figure 8: Examples of colour change after a change in pH (A, B), and shape and colour change after microwave heating (C). Source: Oral et al., 2021.
Although some of the shape change effects of 4D food printing rely to some extent on a 3D printer, other 4D effects, such as change of colour or flavour are based on well understood principles of food chemistry and do not necessarily depend on a 3D printing process. 4D food printing is still at an experimental phase looking for applications beyond curiosity markets. More research in this area may also provide input into solutions for making timed and stimulus-induced changes of food materials a part of food safety monitoring applications. At present 4D food printing is happening mostly in research laboratories and scalability and commercial viability of the explored concepts need to be tested still. Some of the effects studied may find applications outside of 3DFP (Navaf et al., 2022).
3.4 Novel food inks
Since the focus of 3DFP research has shifted in recent years from the shape aspect of printed products in the form of decorative elements mostly printed with chocolate or sugar-based ingredients to healthier ingredients, efforts to develop novel food inks that have explicit health benefits have rapidly increased. However, experimentation with the formulation of food inks based on ingredients of specific nutritional value has shown that these are often difficult to print without additives, hence finding the right combination and proportion of additives that does not affect the ‘healthiness’ of the printed food product is of considerable interest to researchers in the field. Other ‘health enhancing’ effects that might be achieved through 3D printing are based on specific textures that can be achieved with 3D printing and are discussed in the next section.
Desired nutritional enhancements of 3D printed foods are often an increase of protein content in applications such as snack bars, or of ingredients with health benefits, such as fibre or fresh vegetables, which all still pose considerable challenges with respect to printability. A number of proteins have been tested for making protein-rich 3D printed snacks, such as milk protein, whey protein, sodium caseinate, and various plant proteins, such as soy, pea, and bean proteins, mostly by extrusion printing. Binder jetting was used recently successfully to print high protein content structures with a mixture of calcium caseinate powder, starch and medium-chain triglyceride (MCT) powder (Zhu et al., 2022).
This printing method allows for high dry mass content and greater speed and complexity of shapes than extrusion printing. Snacks rich in fibre and protein have been successfully printed using mixtures of various milk and bean proteins including cellulose micro-fibres (Lille et al., 2018). Moreover, as interest in alternative sources of protein for human consumption has increased in recent years the use of insect proteins, such as mealworm powder, and proteins from algae have been tested successfully for 3DFP applications (Azzollini et al., 2018; Bedoya et al., 2022).
Printing with fresh ingredients, such as vegetable purees, has been tested and was anticipated as a way to incentivise children or patients with swallowing difficulties to eat more vegetables. For example, fresh peas, carrots and bok choi with minimal hydrocolloid additives (such as xanthan gum, kappa carrageenan or locust bean gum) could be printed successfully via an extrusion printer (Pant et al., 2021), see figure 9. The Dutch company Gastronology has recently announced to currently up-scale operations and that they plan to be on the market for hospitals and care homes soon with frozen 3D printed foods made from vegetables.
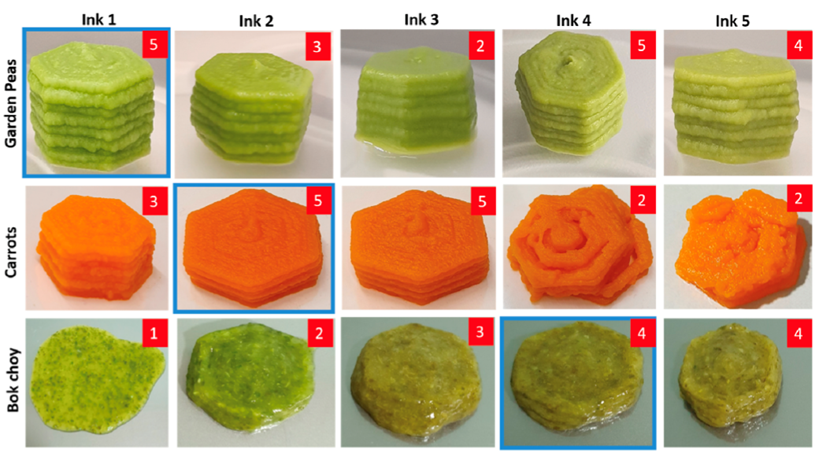
Figure 9: Examples of 3D printed food based on vegetable ingredients (numbers indicate score for shape and print fidelity). Source: Pant et al., 2021.
Other vegetables such as potatoes, mushrooms, yam, spinach and broccoli have been tested either from fresh or from freeze-dried powders with variable success, and some additives, such as starches and gums or gelatine, have to be used in most cases in order to enable printability. Also fruits, such as mango, kiwi, orange, lemon, strawberries have been studied and can be printed mostly with gel-forming printing processes that require the addition of some gelling agent, such as various types of starch or gelatine. 3D printed protein-fortified lemon-based gels have been tested in care homes in a small trial in Denmark (Chow et al., 2021; Waghmare et al., 2022). With respect to adding ‘health enhancing’ ingredients that would turn 3D printed food items into ‘functional foods’ a number of natural bioactive molecule classes, such as carotenoids, polyphenols, vitamins, unsaturated fatty acids and probiotics among others have been studied for their suitability for addition into healthy food inks and for their compatibility with the 3D printing process (Donn et al., 2022; Lu Zhang et al., 2018). Adding such functional molecules does not strictly require a 3D printing process to produce functional foods, however, depending on what type of functional molecule is added, mostly without affecting printability, specific claims can be made about the printed food, such as for example that 3D printed food could help with reducing oxidative stress or positively affect inflammatory conditions, positioning such 3D printed foods close to nutraceuticals, although such claims are still rare (Cotabarren & Palla, 2022). For printing more complex products that combine different nutritional benefits, for example high protein with high fibre content, a number of combinations of proteins and fibre types, for example fava bean extraction fractions, or whole milk powder and wholegrain rye flour have been successfully tested with regards to printability (Johansson et al., 2022; Lille et al., 2018, 2020).
These attempts to find formulations for healthy 3D printed products are still facing many technical challenges, in particular with standardisation and scale-up of recipes, although first attempts to enter niche markets with such health focused 3D printed products are reported to happen possibly in the near future, as mentioned, for example in the Netherlands. Despite various proposed health claims, the actual scientific assessment, and agreement by regulators what needs to be measured to make these claims valid for 3D printed foods is at present still lacking (as for many other foods that are sold with such claims).
3.5 3D food printing for texturising and sensory modification of foods
Textures are as important as flavours in characterising authentic whole foods and 3D printing has in the past five years been explored as a tool to manufacture specific textures as well as for prototyping the sensory experience aspect of certain foods. These attempts were motivated largely by three objectives with consumer products in mind, namely to achieve dietary change by modifying sensory experience, to create microstructures that can mimic natural textures, for example in the plant based and in-vitro meat sectors, and to provide texturised foods for patients with swallowing difficulties.
3.5.1 Dietary objectives
A change of sensory or taste experience was achieved for example by changing infill pattern and infill density of 3D printed foods to change sensory perception in order to create the “sensory illusion” of greater sweetness or saltiness, despite less salt or sugar being used with the ultimate aim to help consumers reduce these ingredients in their diets (Fahmy et al., 2021; Zhu et al., 2020). Also the contribution of microstructure and resulting chewiness in connection with the sensation of satiety, have been explored (Lin et al., 2020). These 3D printing applications may in the future also serve as exploratory tools for food product design and prototyping, rather than being used as production methods.
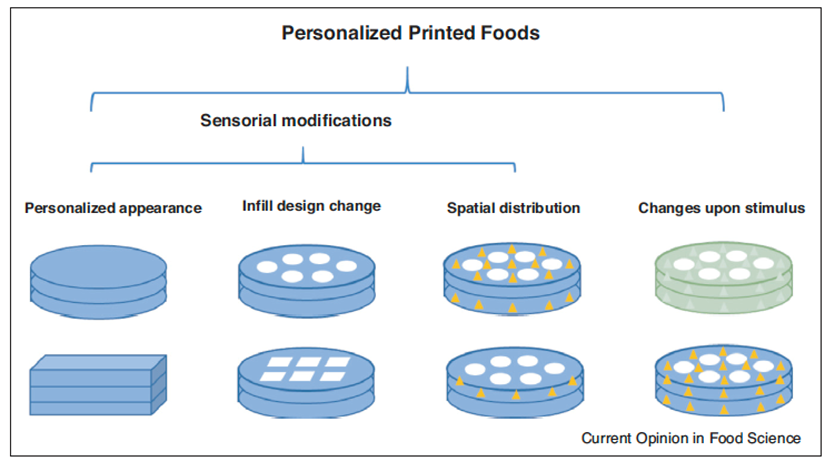
Figure 10: Examples of textural design concepts for sensory modification using 3D and 4D printing. Sensory experience can be changed with different infill patterns and spatial distribution of ingredients, including 4D effects upon external stimuli. Source: Ma & Zhang, 2022.
3.5.2 Mimicking natural textures
One of the initial weaknesses of the plant-based and cultured meat alternatives products has been a lack of convincing texture. After initial experimentation with the addition of ‘texturizing’ ingredients into the ingredient mix both plant based and cultured meat alternative producers have in the last three years started to use 3D printing approaches to texturise their products (K. Handral et al., 2022). One of the successful plant-based meat companies in Europe, Novameat, Spain, has developed standard 3D printing technology further into a patented micro-extrusion appliance that can generate meat like textures. Likewise, Steakholder foods, formerly MeaTech 3D, Israel, use a modified 3D printing approach for the same purpose to produce their cultured meat products including more recently fish. Revo foods, Austria, (formerly Legendary Vish) use allegedly 3D printing to produce plant-based seafood products with their salmon products already available in some Austrian supermarkets as well as through local delivery services. They use pea proteins, algae and plant oils as ingredients, making their products a source of omega 3 oils. Revo has recently allegedly entered markets in 20 countries including the UK and signed contracts with REWE, one of the largest food retailers in Europe (Vegconomist, 2022a, 2022b). However, industry insiders doubt that the used technology would strictly classify as 3D printing.
3.5.3 Creating textured food for dysphagia patients and the elderly
Texturising foods with 3D printers for patients with swallowing difficulties due to neurodegenerative and other illnesses, called dysphagia has been explored by a number of researchers (Kouzani et al., 2017). Limited real-world tests have been carried out meanwhile, also driven for a while by an EU project titled: Personalised Food for the Nutrition of Elderly Consumers (PERFORMANCE) that started already in 2014 coordinated by the German company Biozoon Food Innovations, and the German government has supported research into testing such products in care homes. However, updates or publications on outcomes from this initiative are not available at present. Textures produced for dysphagia patients via 3D printing, using vegetable and meat based ingredients have been shown to fulfill food consistency requirements of the International Dysphagia Diet Standardization Initiative (IDDSI), framework and the state of the art of 3D printing for dysphagia patients has been reviewed very recently (Liu et al., 2021; Lorenz et al., 2022). Like other advanced applications for food printing, printed food for patients or the elderly is currently not available beyond a few small proof-of-concept trials that have been carried out in the past few years.
Revision log
Published: 3 March 2023
Last updated: 5 March 2024