Introduction: 3D printing technologies in the food system for food production and packaging
This introduction is a top-level overview of the field of 3D food printing (3DFP) focusing on its historical evolution and current trends that may impact on the food system, providing the wider context within which 3D printing is analysed in this report, with areas of importance for the FSA remit highlighted.
This introduction is a top-level overview of the field of 3D food printing (3DFP) focusing on its historical evolution and current trends that may impact on the food system, providing the wider context within which 3D printing is analysed in this report, with areas of importance for the FSA remit highlighted. Due to the limited amount of information available on the current use of 3D printing technologies for the production of food packaging, this topic is presented separately in chapter 4 of this report.
1.1 The context of 3D printing
3D printing, also called additive manufacturing, represents a range of technologies that creates 3D objects through a layer-by-layer deposition process using digital image files, usually generated in Computer Aided Design (CAD) software. As 3D printing has evolved over the past four decades, a number of technical additive manufacturing concepts have matured into robust individual technologies, as defined by the American Society for Testing and Materials (ASTM) International Committee F42 on Additive Manufacturing Technologies. These are at present: vat photopolymerisation, powder bed fusion, material extrusion, material jetting, binder jetting, directed energy deposition and sheet lamination (ASTM International, 2022). Commercially available printers deploying these engineering concepts for specific applications and materials have found their places in various industries, each with their own strengths and weaknesses and at very different price points. Despite this variety of technical printing approaches the currently most widely used 3D printers, also in consumer markets, deploy a form of Fused Deposition Modelling (FDM), sometimes also referred to as Fused Filament Fabrication (FFF), based on extrusion of a thermoplastic material that hardens after being deposited through a heated filament nozzle. With regards to the material deposition process, FDM/FFF is an extrusion-based printing method, as opposed to other methods that build structures for example via photopolymerisation of liquid substrates, or fusion of powder particles. Generally, 3D printing is considered today a valid technology where highly customised and bespoke products need to be made in small numbers, often in a decentralised fashion, such as in the case of spare parts in remote locations, as it saves the costs and time of engineering a specific manufacturing process to make the product as well as supply logistics. Moreover in design, art and fashion 3D printers have found their place for creating unique complex designs (Gebhardt et al., 2018; Shahrubudin et al., 2019).
After a slow evolution over the past four decades 3D printing has now reached a level of maturity that it enables viable commercial applications at scale in a number of industries. While early applications of 3D printing were focusing on complex shapes and rapid prototyping, the technology can today deliver in some areas a reproducibility and quality of final products such that it is now considered an industrial manufacturing process in its own right. From buildings to tissues assembled from human cells, a wide range of input materials such as polymers, including bio-polymers and various resins, metals and ceramics among others can be used today for the production of complex structures using 3D printing technologies (Derossi et al., 2021; Patra & Young, 2016; Tay et al., 2017).
Associated with these capabilities, new business models have been developed making 3D printers and 3D printing services and products available to wider consumer and B2B markets. However, while for some lower-throughput applications 3D printing can today replace other production methods, it is also acknowledged that it is on the whole still a slow performing technology with well understood limitations, and where alternative methods in particular for mass production exist, 3D printing would in most cases still not be cost effective, or able to deliver the consistency and quality of final product required (Gebhardt et al., 2018).
1.2 3D printing of food – past evolution
The concept of producing food via some digitally operated machine is often traced back to science fiction novels and the “replicator machine” featured in the 1960s original Star Trek series. The earliest attempts to seriously propose engineering solutions for 3DFP go back to around 2001 when Nanotech Instruments Inc. filed a patent for a 3D fabrication method to produce customised birthday cakes, however without at the time building a physical prototype of the suggested device.
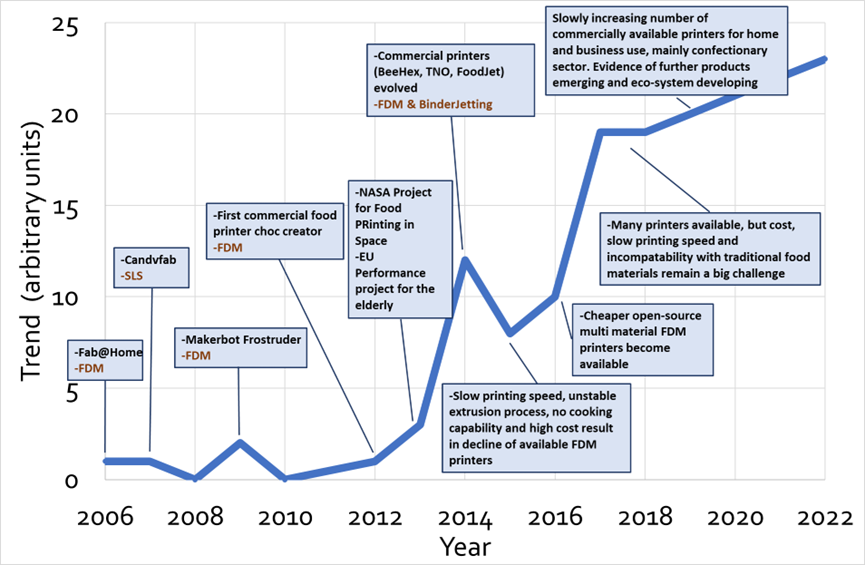
Figure 1: Overview of the temporal evolution of 3DFP (modified from: Agunbiade et al., 2022).
Throughout the mid to late 2000s kitchen appliances producers, such as Electrolux and Philips with its Food Creation Printer translated such concepts by testing the printing of food items from a number of ingredients, stored in cartridges and deposited in layers via a robotic arm, including the development of a graphical user interface that enabled control of ingredient proportions and shapes. The Philips product was presented as market ready in 2008 and gained media attention by being used by a few at the time popular ‘molecular gastronomists’, without however being developed further to enter wider consumer markets. Around the same time the first patents explicitly proposing additive manufacturing technologies for printing food ingredients were filed (Baiano, 2022; Pérez et al., 2019; Portanguen et al., 2022). The idea of printing food also gained attention as NASA has been exploring food printing concepts for space missions since around 2005 and later launched its ‘Advanced Food Technology Program’ in 2013 with the aim to make food printing feasible for space travel. However, almost a decade on, several technical issues are still unresolved for space applications (Enfield et al., 2022; Long‐zhen Zhang et al., 2022).
Besides appliances manufacturers, prominent academic institutions have been driving technology innovation in food printing conceptually, such as MIT with its Virtuoso Mixer, Digital Fabricator and Robotic Chef (2010), or the Creativemachines Lab at Columbia University where food printing has been explored and tested since 2005 starting with their Fab@Home project and continuing subsequent research, up to recent systems implementing printing devices with integrated cooking functionality using lasers (Creativemachines Lab, 2022; Everett, 2021). Many other academic institutions have over the past decade delivered solutions for specific food ingredients, driving the diversification of specific application areas. For example, University of Exeter (UK) spinout Choc Edge Ltd has developed and commercialised the first UK chocolate printer in 2012 and tested their products for some years, but the company was dissolved in 2021.
After the first technical articles published in scientific journals around 2010, such as the original work on printing chocolate by Liang Hao and co-workers at University of Exeter (Hao et al., 2010), the first widely referenced review articles by pioneers in the field surveying 3DFP research with regards to challenges and opportunities appeared around 2015 (Lipton et al., 2015; Sun et al., 2015; Wegrzyn et al., 2012). At his point, specific opportunities for health applications of 3DFP were explicitly formulated and the first comprehensive text book on the subject has been published in 2018 (F. Godoi et al., 2018; Lipton, 2017). Over the past five years publications have grown almost exponentially (although starting from low numbers, see section 5.1.1) and an industry for producing academic reviews on 3DFP has emerged, sometimes of questionable quality due to repetitive information and a lack of clear research focus and novel insights.
More recently, collaborative networks of academic research institutions and commercial players focusing on 3DFP have emerged. One European example is The Netherlands Organisation for Applied Scientific Research (TNO), which supports development of various innovative food printing applications from plant based meat to healthy products for children and patients as part of the Dutch Digital Food Processing Initiative in a collaboration with Wageningen University & Research and Eindhoven University of Technology. TNO holds patents in 3DFP and has also been involved with developing the 3D pasta printing technology for the Italian pasta maker Barilla, which has since offered 3D printed pasta through its subsidiary BluRhapsody. Other larger research groups conducting fundamental research into 3DFP also exist in the UK, Canada, Spain, Italy, Finland, Germany, Australia and Singapore (see section 5).
1.3 Definition of 3D food printing
The wider definition of 3D printing as technologies that can create 3D objects through a layer-by-layer deposition process using digital image files applies to most 3D printed food items. Definitions in the academic literature mostly focus on the printing process itself defining 3DFP as a process of layered deposition of food ingredients to form edible 3D structures (Baiano, 2022; Derossi et al., 2021; Portanguen et al., 2022). However, one needs to consider that the printing process of food often cannot be separated from the pre- and post-processing steps that are essential for generating the actual food item as shown in figure 2 (Demei et al 2020).
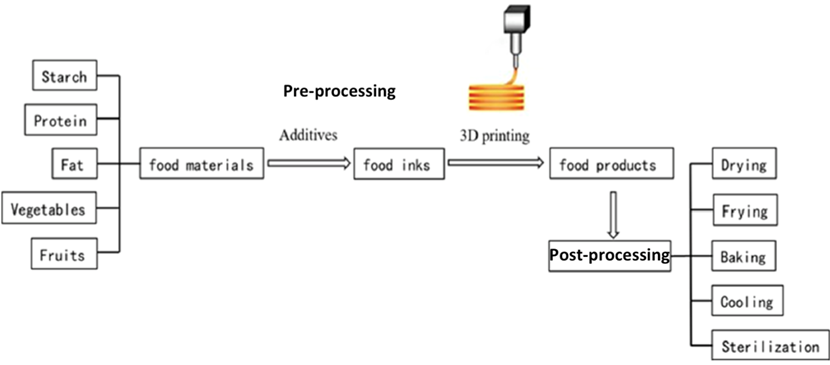
Figure 2: Workflow of a typical 3DFP process using extrusion printing, the most widely used printing technology (modified from Demei et al., 2020)
In contrast to the most common inorganic and organic materials that are used for general 3D printing applications, such as polymers, or metals, the ingredients for food printing are mostly not stable and inert, and can also have a large degree of variation in their material properties, even when using the same recipe (batch to batch variation of food ingredients is a common issue in gastronomy cooking). However, preparing the right ingredient composition for the printer in question is in most cases a precondition for the successful operation of the printer. This is particularly crucial for complex mixtures of ingredients. Likewise, many 3D printed food types require time-critical post processing to make the printed product edible. Hence, in particular from a food safety perspective, it needs to be considered when defining a 3D printed food item whether pre- and post-processing should be considered as integral parts of the printing process, or whether for example final post-processing steps can be completely independent from the printing process, as is the case for example for 3D printed pasta that is frozen after printing and then sold to consumers.
What processing steps are considered intrinsic to the 3D printing process also needs to be clearly defined as currently printing devices emerge that integrate a simultaneous mixing step or a cooking step for example with lasers, or ohmic heating for pre-baking of cake batter while printing (Khodeir et al., 2021). A widening of the definition might also be necessary in order to be able to make distinctions, such as whether specific 3D printed food products would fall into the category of ‘highly processed foods’, or not. Moreover, integrated cooking, or other post processing steps may impact the assessment of 3D printed food with regards to their shelf-life or impact on supply chains etc.
Given these considerations it might be necessary to explicitly include pre- and/or post-processing aspects of the technology in the definition of 3DFP. For the purposes of this report, whenever we use the term 3DFP we mean such a wider definition that could be formulated as:
‘3DFP comprises technologies that can create 3D objects intended for human consumption through a layer-by-layer deposition process using digital files, including pre- and post-processing steps that are essential for producing the final product’.
1.4 Current state of 3DFP - overview
Despite clearly increasing research and commercial activity around food printing over the past 15 years, and in particular the past five years, only a small number of 3D printed food types have matured from the conceptual prototype stage to commercially available and viable products, and most of them only in limited numbers in the context of semi-commercial startup settings, such as chocolate and sugar-based ingredients, food for the elderly and patients with difficulties swallowing, cultured (in-vitro) and plant-based meat, pasta as well as dough-based baked foods, and cheese.
The temporal evolution and scale of technology innovation in 3DFP driven by academic and industry research is well documented in publication and patent data on 3DFP as shown in sections 5.1.1 and 5.1.2 and some comprehensive reviews of the existing literature on 3DFP have been published recently (Baiano, 2022; Derossi et al., 2021; Isabel Diañez et al., 2022; Portanguen et al., 2022). From these sources it is very apparent, that despite some earlier concept development the technology has mainly evolved over the past decade at a small scale and slow pace, with very few active commercial players. Analysis of patent and academic literature data also show that currently only a limited number of food types, mentioned above, are currently printable and technical improvement and process adaptation to different food types is the main concern of most research activity at present.
Very recent innovations, such as 4D printing applications for food in which the texture, colour, taste, or shape of the printed item change over time, or upon external stimuli, are at an early stage and need to become still more robust and cost effective before they might offer added value and can enter wider consumer markets.
However, over the past five years, academic research into the technical possibilities and challenges of food 3D and 4D printing, as well as media reporting on the technology has rapidly increased, and a number of 3D food printers for different types of food, 3D-printed food products as well as service offerings have reached limited consumer and B2B markets (Jayaprakash et al., 2020). In addition, several emerging niche applications for printed food are now specifically promoted by commercial players, such as for printed personalised nutrition, for example Getnourished UK, or for texturizing foods for patients with difficulties eating regular food in hospitals and care homes (Lorenz et al., 2022). 3D printing as a possible solution in this context was already published on the European Commission website in 2014, and research on 3D printing as a technology to improve the eating experience for dysphagia patients in care homes has been tested for example in Germany by government supported research. However, larger commercial operations offering these services at scale are still not on the market.
Despite increasing research and exploration of such potential application areas, 3D and 4D printing of food is at present still a curiosity niche phenomenon, most advanced possibly in the fine dining and confectionery segment for the production of edible and sometimes personalised decorative food items. However, current maturity levels of the few commercially operating food printers, some service offerings on the market, and continuous low-level engagement of large food producers with the technology, are indicative of the potential of 3DFP to be able to reach wider consumer markets via different channels in the future.
1.5 Potential wider impacts of 3D food printing
Anticipating regulatory responses, producers of 3D printers for food have already voluntarily implemented food-compliant industry standards, as for example BASF in Germany has obtained food-compliant TÜV certification for its parts being used in food 3D printers, and food printer suppliers specifically advertise which of their printer parts comply with food grade certification (Molitch-Hou, 2021).
Wider systemic impacts of 3D printing of food are discussed in the academic literature and diversification of the technology into specific sub-niches indicate that 3D printing might act as an enabling or supporting technology for other technology-enabled trends in the food system, such as personalised nutrition, alternative proteins, use of food side- or waste streams (such as in the seafood industry that has in Asia traditionally used seafood production waste for making Surimi paste that can be shaped in different ways and sold as a product in its own right), plant based meat alternatives, cultured meat, functional foods and health nutrition. Moreover, food printing currently appears to be evolving from a focus on the shape aspect of printing towards finding solutions for real food processing challenges and novel ways to for example increase healthy ingredients such as fibre, or reduce salt, sugar and fat intake via printing of specific textures that modify the sensory experience of food, and an area that could be described as food product prototyping (Ma & Zhang, 2022; Portanguen et al., 2022). In addition, some claims about the future potential impacts of 3DFP around its ability to possibly increase sustainability of food production in some cases, or that it might enable business models of decentralised food production affecting supply chain models have been made repeatedly in the academic literature, although without presenting any evidence for these claims (León‐Bravo et al., 2019; Verma et al., 2022).
These recent developments indicate that the technology might be entering the food system via different routes and it is therefore important for the FSA to understand potential risks and opportunities of the technology, and in particular whether any regulatory intervention might be required with regards to its remit ensuring that food is safe to consume, food is what it says it is, and food is healthy and sustainable, as stated in the Food You Can Trust FSA Strategy 2022-27. Given the novelty of food 3DFP and its recent dynamic R&D development, the FSA needs to understand what the potential issues of the technology might be with regards to its remit.
1.6 Objectives
This report is intended to assist the FSA in policy development and regulatory decision-making relating to 3D printing of food and food packaging by:
1. Providing an overall assessment of 3D printing technologies as used in the current food system.
This objective aims to explore the current state of 3D and 4D printing technology used in food applications, including 3D printing of food packaging, followed by mapping the potential technological developments on the horizon for the next decade. The findings will be covering both current and emerging research as well as existing applications in the industry.
2. Establishing to what extent developments in 3D and 4D food printing will impact the food system and may act as a catalyst for further change, including shifts in manufacturing and supply of food as well as in consumer trends.
A thorough review of current and emerging technology advances in the context of commercialisation, markets, supply and demand as well as consumer trends will enable identification of wider contexts within the food system that might be of relevance to FSA when assessing impact of food 3D printing on consumers with regards to FSA remit.
3. Establishing implications of 3D printing technologies for food safety for consumers and the food system overall as well as their potential benefits.
Given identified trends and drivers affecting the further evolution of 3DFP, this report will provide an analytical framework and recommendations for the FSA to stay abreast of developments and be able to act when required by designing relevant policy to fulfil its remit.
1.7 Research questions
This report will address a number of relevant research questions to establish the current state of 3DFP, from a technical as well as market and food safety risk perspective, what the trends and drivers and potential future developments of 3DFP may be, and how the technology might affect different parts of the food system and consumers. The current maturity level of 3DFP is at an early stage but has to date generated sufficient evidence to allow an assessment of its potential impact on several aspects of the UK food system. Hence, the following research questions will be addressed:
- What is the current state of the market for the use of 3D printed food items offered by food industry players (service, industry, retail) and what B2B and consumer markets make use of 3D food printers for which purposes?
- What is the state of the technology today, what is its potential over the next 10 years, and which specific markets might have the biggest potential for growth?
- What are the most prevalent offerings and what market segments are they entering?
- How might 3DFP technologies impact the traditional food value chain, and what cross cutting synergies of the technology might impact other trends, such as personalisation of nutrition/food, distributed food production, use of 3D printing in the production of lab-grown meat, or the use of alternative and novel proteins?
- What are potential barriers and contextual factors that may impact adoption and what are technical challenges to the technology?
- What impact might 3DFP have on food safety, and what claims are made by producers about 3D printed food?
- What are the impacts on vulnerable groups, such as patients and the elderly who might be offered 3D printed food in hospitals and care homes without being able to make an informed decision about its consumption?
- What are the potential risks to consumers of 3D printed food packaging, or packaging materials used to package 3D printed foods in line with the above research questions.
1.8 Methodology
This report is based on literature review and standard rapid evidence assessment methodologies used for open access information in academic and grey literature, and no primary research was carried out. The literature review, data gathering and report preparation was conducted in the following phases:
Phase 1: Broad review of academic and grey literature as well as patent databases using custom web search and data analytics tools using a first set of key words and search strings based on the field of enquiry, 3DFP.
The main data source for the initial academic literature search was the Lens database.
Patent searches were conducted using the main IP offices worldwide covering the past 10 years:
- WIPO (patentscope)
- the World Intellectual Property Database
- EPO (Espacenet)
- the European Patent Office
- USPTO, the US full text patent database Additional grey literature was reviewed through researcher-led search
An initial review and analysis of the data was conducted mainly aiming at refining the keywords and search strings
Phase 2: A refined set of keywords and search strings was used to repeat searches. The resulting data was analysed in detail to identify key areas for further research, refine the keywords and search strings further and generate the first data set to be used as basis for human based deep analysis for gap and noise identification
Phase 3: A final set of keywords and search strings was used to conduct searches and the resulting literature was subjected to rigorous researcher-led deep analysis. At this stage, noise was removed from the data, all figures and graphs were generated, reviewed and final outputs produced.
In this way the most relevant literature was identified and used for creating the report content. In addition, relevant social media sites were analysed to get a sense of consumer sentiment and prevalence on the topic of 3DFP. This exercise was not algorithm-based sentiment analysis, but researcher-led analysis on subjects discussed in social media with regards to 3DFP. Throughout the research we benefited from discussions with our expert consultant Dr Lu Zhang, Wageningen University & Research, the Netherlands.
Phase 4: All information was iteratively analysed and verified by different researchers before integration into the draft report. The draft report was sent to both the expert consultant and FSA representatives for review and comments.
Phase 5: Comments and input from both FSA and the expert consultant were considered and addressed. The report was then finalised and prepared in the required format for submission.
Hanes diwygio
Published: 3 Mawrth 2023
Diweddarwyd ddiwethaf: 5 Mawrth 2024
Hanes diwygio
Published: 3 Mawrth 2023
Diweddarwyd ddiwethaf: 5 Mawrth 2024