Rapid Risk Assessment: What is the risk from microcystins in the edible flesh of fish caught from Lough Neagh?
Risk question: What is the risk from microcystins in the edible flesh of fish caught from Lough Neagh?
During the Summer and Autumn of 2023, Lough Neagh in Northern Ireland was affected by a cyanobacterial bloom. Testing of water from within the bloom reported high concentrations of one type of cyanobacterial toxin, microcystins.
Samples were taken from the Lough of eels, roach, perch, pollan and bream and tested for a range of cyanobacterial toxins, including microcystins, nodularins, anatoxin, cylindrospermopsin and saxitoxin. Each sample comprised 10 fish, and five samples were taken of each species, except for bream for which a single sample was collected. The fish were dissected and the edible flesh, intestine, liver, roe, gonad and/or gills analysed separately. Microcystins were detected at a range of concentrations in the various parts of the fish that were sampled - intestine, liver, roe and/or gills, but were not detected in the edible flesh of any of the fish samples. Averaged across the samples, the highest concentrations of microcystins were quantified in the intestine samples, followed by the liver samples, with low concentrations were detected in the gills and a small number of the samples of gonads and roe. None of the other toxins were detected in any fish sample.
The initial analysis for microcystins was of free toxins only. However, there is evidence that microcystins which are covalently bound to proteins are also bioavailable and therefore 22 fish tissue samples, including nine fish flesh samples, were also sent to another laboratory where they were analysed by a method which measures the total concentrations of microcystins, free and protein-bound. The viscera tissue samples chosen for the further analysis were those with the highest concentrations of the free toxins, while the fish flesh samples included 2-3 samples each of eels, roach, pollan and perch. The concentrations of total microcystins found in viscera samples were around one order of magnitude higher than the concentrations of free microcystins that had been measured. However, microcystins were still not detected in the edible fish flesh samples.
It is possible that microcystins were not present at any level in any of the fish flesh samples. However, the presence of microcystins in the edible flesh of fish has been reported in the scientific literature, albeit at lower levels than those in the gastrointestinal tract or other parts of the viscera such as liver (Testai et al., 2016). Since microcystins were detected in other parts of the fish sampled from Lough Neagh it is also possible that they were also present in the fish flesh but at levels below the limits of detection of the analytical methods. The limit of detection of the analytical method for total (free + bound) microcystins was 10 µg/kg wet weight.
An upper bound dietary exposure assessment was conducted. While a lower bound exposure assessment would assume the microcystins were not present in the edible flesh, i.e. a concentration of 0 µg/kg, the upper bound approach assumed they were present at the limit of detection of 10 µg/kg. The true concentrations may be between these levels. The exposure assessments consider high consumers of fish (97.5th percentile). For eels, consumption data were used from the National Diet and Nutrition Survey (NDNS). For roach, perch, pollan and bream. No consumption data were available from the NDNS and consumption data for trout were used instead as a proxy.
The main target organ for toxicity of the microcystins is the liver, though other organs may also be affected. The microcystin most studied toxicologically is microcystin-LR, which is one of the most common microcystins. A WHO review established a provisional tolerable daily intake (TDI) for microcystin-LR of 0.04 µg per kg bodyweight (bw). WHO recommended that exposures to total microcystins should be compared to this provisional TDI, though there is uncertainty with this as individual microcystins are likely to differ significantly in their toxic potencies.
Estimated dietary exposures of total microcystins were all within the provisional TDI, indicating no health concern from consuming the edible flesh of these species.
Since fish may be caught and prepared for consumption not only by food business operators but by recreational anglers, concern has been raised that evisceration may be incomplete or the edible flesh may become contaminated in the process, and therefore this was also considered in the risk assessment. This was based on the sample of fish which contained the highest concentration of total microcystins in a viscera component, which was a sample of roach with a particularly high concentration of microcystins in intestine. It was assumed that 10% of the relative proportion of intestine to flesh in the fish would be inadvertently consumed with the flesh. In this scenario, dietary exposures would be within the provisional TDI in most age groups or would marginally exceed the TDI, but this would not be toxicologically significant. In addition, since this exposure scenario used an upper bound approach to the concentration in flesh, and used the highest concentration in any viscera sample, it is not clear that there would be any exceedance of the provisional TDI in practice. Overall, it appears unlikely that consumers will substantially exceed the provisional TDI on a long-term basis due to incomplete evisceration of fish.
Overall, exposure to microcystins from eating the edible flesh of the tested fish species would not be expected to cause adverse effects in consumers, including if the fish is inadequately eviscerated. Therefore, we consider the frequency of adverse reactions in the general population to be negligible, so rare that it does not merit to be included.
Based on the possible levels of exposure to microcystins from fish from Lough Neagh, it is considered that any liver injury, were it to occur in consumers of fish, would result from long term exposure and be mild. Overall, we consider the severity of illness that could potentially occur as a result of exposure to microcystins from consuming edible fish flesh from Lough Neagh to be medium (i.e. moderate illness, incapacitating but not usually life-threatening and of moderate duration).
We consider the level of uncertainty to be medium (i.e. there are some but no complete data available), but that this does not affect the conclusion of the risk assessment since many of the key uncertainties are addressed within the risk assessment. However, future monitoring would be useful to assess whether microcystin concentrations in the fish change over time.
Following testing of samples of water from the Lough and of eels, roach, perch, pollan and bream, a number of cyanobacterial toxins were not detected in either the water or fish (nodularins, anatoxin, cylindrospermopsin and saxitoxin). However, microcystins were detected in water samples and in various parts of viscera in fish, though not in the edible flesh. This risk assessment has been produced to consider the risks from eating the edible flesh of fish.
It has been assumed that:
- Cyanobacterial toxins which were tested for but not detected in any sample of fish, nor in water samples from the Lough which were contaminated by cyanobacteria, were not present at any levels in fish flesh
- Microcystins, which were measured in water samples from the Lough, and in fish viscera samples but were not detected in edible fish flesh, may be present in fish flesh at levels below the limit of detection of the analytical method
- The concentrations of microcystins present in samples of fish taken in Autumn 2023 are reflective of long-term levels in fish flesh and therefore long-term dietary exposure
This risk assessment also includes a consideration of the potential risks if evisceration of fish is inadequate or incomplete.
The Science, Evidence and Research Division of the FSA produced initial technical advice on cyanobacteria and cyanotoxins in fish. The majority of available data on cyanotoxins in fish affected by cyanobacterial blooms related to microcystins, which can cause liver damage. They are mostly found in the gastrointestinal tract of fish but can also accumulate in the liver. Microcystins can also be present in fish muscle, but it was highly uncertain how the concentrations in edible flesh would relate to concentrations in the water and the prey and other feed of the fish.
The FSA produced risk management advice for businesses and consumers. Fish that were dead, or show any signs of sickness, in the area of a bloom should not be caught or eaten. The risk could be managed by removing the parts that the toxins accumulate in, especially the liver. Food businesses may be able to manage the risks, but there was an increased risk to consumers preparing and handling fish in the home.
The FSA worked with the Centre for Environment, Fisheries and Aquaculture Science (Cefas) to develop sampling and testing of water samples from within cyanobacterial bloom in the Lough and of fish from the Lough for cyanobacterial toxins. Brown trout were not currently being fished, but samples were taken of eels, roach, perch, pollan and bream. These include planktivorous species, which are reported to accumulate higher concentrations of cyanobacterial toxins than carnivorous species (Falfushynska et al., 2023).
Each sample comprised 10 fish, and overall five samples were taken of each species, except for bream for which a single sample was collected. The most frequently reported and studied cyanobacterial toxins in freshwater cyanobacterial blooms are the microcystins. However, it was agreed to test for a range of cyanobacterial toxins for which methods were available. The cyanobacterial toxins tested for included microcystins, nodularins, anatoxin, cylindrospermopsin and saxitoxin. The microcystin analyses were of the free toxins only and did not include protein-bound toxin. Therefore, Cefas also sent a number of subsamples of the same samples to another laboratory, which had a method to analyse total free + protein-bound microcystins in fish.
Microcystins were measured at high levels in the water samples. The other cyanobacterial toxins were not detected. The fish were dissected and the edible flesh, intestine, liver, roe, gonad and/or gills analysed separately. Free microcystins were measured at a range of concentrations in various of the fish samples in intestine, liver roe and/or gills. No microcystins were detected in the edible flesh of any of the fish samples. None of the other toxins were detected in any fish sample.
A total of 22 fish tissue samples, including nine fish flesh samples, were analysed for total (free + protein-bound) microcystins. The viscera tissue samples chosen for further analysis were those with the highest concentrations of the free toxins, while the fish flesh samples included 2-3 samples each of eels, roach, pollan and perch. Concentrations of total microcystins in viscera samples were approximately one order of magnitude higher than the concentrations of free microcystins. However, no microcystins were detected in any of the edible flesh samples.
While microcystins were not detected in any of the edible flesh samples, microcystins have been reported in the scientific literature to occur also in fish flesh, at lower levels than in intestine or liver. Therefore, it is possible that microcystins were present in edible flesh at levels below the limits of detection for the analytical methods.
One of the most common microcystins is microcystin-LR (MC-LR), though over 250 microcystins have been identified (WHO, 2020). MC-LR is also the microcystin that has been most studied toxicologically and is amongst the most potent.
The main target organ for toxicity of the microcystins is the liver, though other organs may also be affected (WHO, 2020). MC-LR did not induce gene mutations in bacterial cells or chromosome aberrations in mammalian cells in vitro, although an increased frequency of polyploid cells was observed in mammalian cells which indicated that it may be aneugenic (IARC, 2010). However, neither MC-LR nor cyanobacterial extracts increased micronucleus formation in cultured human lymphocytes, indicating neither clastogenic nor aneugenic effects (Abramsson-Zeterberg et al., 2010). Evidence suggests that microcystin-LR may act as a tumour promotor in the liver and possibly other tissues (WHO, 2020; IARC, 2010). MC-LR is classified by the International Agency for Research on Cancer (IARC) as possibly carcinogenicity to humans (Group 2B) based on studies in rats and mice in which it promoted the development of pre-neoplastic lesions (IARC, 2010). Developmental toxicity studies in mice did not identify adverse effects. A number of studies reported adverse effects on male and female reproductive organs. However, these studies mainly used intraperitoneal dosing which can lead to much higher internal exposures than would be achieved by oral dosing, and several more recent studies which used oral dosing had methodological and reporting deficiencies (WHO, 2020). Therefore, further reproductive toxicity data would be required to confirm the adverse effects and identify dose-response relationships. Only limited data are available on potential neurological, immune and haematological effects.
The mode of action is inhibition of protein phosphatases, resulting in destabilisation of the cytoskeleton and microtubules (WHO, 2020). This results in altered cellular function, followed by apoptosis and necrosis. At low doses, inhibition of the protein phosphatases results in cellular proliferation, hepatic hypertrophy and tumour promotion activity.
Key studies in laboratory animals
For full reviews of the toxicology data on microcystins see Testai et al. (2016) or WHO (2020). The following summarises key studies of value for risk characterisation.
Fawell et al. (1999) administered microcystin-LR by oral gavage to groups of 15 male and 15 female mice at dose levels of 0, 40, 200 and 1000 µg/kg body weight (bw)/day for 90 days. All mice were examined daily for signs of clinical toxicity. Bodyweights and food consumption were measured weekly, and eye examinations were conducted at the start and end of the study. Blood samples were taken during the final week for haematological and clinical chemistry analyses.
Histopathological analyses were conducted on all tissues from control and high dose group animals, and on the lungs, liver and kidneys from the other dose groups, with particular focus on any gross lesions observed at necroscopy. Histopathological changes were only observed in the liver, and were reported to be multifocal minimal/slight chronic inflammation with deposits of haemosiderin and multifocal single hepatocyte degeneration throughout the liver lobule. These were mainly observed in the high dose group with less marked lesions in smaller numbers of animals in the mid-dose group. There were no changes observed in the low dose or control groups. Haematological changes were limited to small but significant increases in mean haemoglobin concentration, red cell counts and packed cell volume in females in the high dose group.
A number of changes in blood chemistry parameters were observed in the mid and high dose groups, including high plasma alkaline phosphatase levels in both sexes at the top dose, raised transaminases in both sexes at the top dose and in males at the mid-dose, and reductions in plasma albumin and total protein levels in males only at the mid and high doses. The no-observed-adverse-effect-level (NOAEL) was concluded to be 40 µg/kg bw/day.
Heinze (1999) administered doses of 0, 50 and 150g/kg bw/day microcystin-LR to groups of 10 male rats via their drinking water for 28 days. Relative liver weights were increased by 17% and 26% in the low and high dose groups, and absolute liver weights were also reported to be increased. Liver lesions were observed in both treatment groups, with slightly greater severity in the high dose group. Levels of alkaline phosphates (ALP) and lactate dehydrogenase (LDH) were increased in both treatment groups, while there were no changes in alanine aminotransferase (ALT) or aspartate aminotransferase (AST). No NOAEL was identified from this study. The low dose level of 50µg/kg bw/day is therefore the lowest observed adverse effect level (LOAEL).
Ueno et al. (1999) conducted a chronic toxicity study in which groups of 20 six-week-old female BALB/c mice were administered microcystin-LR in their drinking water at concentrations of 0 and 20µg/L for 18 months. There were no clinical signs of toxicity or treatment-related effects observed on survival, body weight, food or water consumption, haematology or histopathology. A statistically significant increase in serum cholesterol (22%) was observed at month 18, but not months 3, 6 or 12. The toxicological significance of this was considered uncertain as it was a single finding and not associated with any other treatment-related changes. In addition, immunohistochemical analysis did not indicate hepatic MC-LR accumulation. The 20µg/L group was stated to have received a total dose over the 18 months of 35.5µg/mouse. Based on the adult mean body weight reported of 26.68 g and the 567 days of dosing, this is approximately equivalent to a dose level of 2.3µg/kg bw/day (WHO, 2020).
Fawell et al. (1999) conducted a developmental toxicity study of microcystin-LR in mice. The mice were dosed by oral gavage at 0, 200, 600 or 2000µg/kg bw/day on days 6-15 of gestation. The dose level of 2000µg/kg bw/day was selected as it had been shown to cause maternal toxicity in a small dose range finding study, and the dose level of 200µg/kg bw/day was selected as being the likely NOAEL for maternal toxicity. Substantial maternal toxicity was observed at the top dose, including deaths of 7/26 dams and two being humanely euthanised. These dams also showed macroscopic changes to the livers. Surviving dams showed no clinical signs or effects on food consumption or body weight. Fetal body weight was reduced compared to controls and delayed skeletal ossification was observed. However, there was no evidence of embryolethality, and the numbers of implantations and live fetuses were unaffected. No treatment-related increases in the incidence of major or minor external, visceral or skeletal fetal abnormalities were apparent. There were no maternal or developmental effects apparent in the low or mid-dose groups and therefore the NOAEL was 600µg/kg bw/day for both maternal and developmental toxicity.
Key human data
An outbreak of acute liver failure occurred at a dialysis clinic in Caruaru, Brazil in 1996. The dialysis water was found to be contaminated with microcystins and cylindrospermopsin, and the microcystins were considered likely to be the major factor, specifically microcystin-YR, microcystin-LR and microcystin-AR (Carmichael et al., 2001). Out of 131 patients treated, 116 experienced visual disturbances, nausea and vomiting, and subsequently 100 developed acute liver failure, of which 76 died. Analyses of liver samples from 39 of the patients who died identified the presence of microcystin-YR, microcystin-LR and microcystin-AR, and the mean concentration of total microcystins was 223 ng/g. This was compared with a concentration of 125 ng/g microcystin-LR measured in the livers of mice dosed with a lethal dose of microcystin-LR by intraperitoneal injection.
A second event occurred at a Dialysis clinic in Rio de Janeiro, Brazil in 2001. A survey identified a microcystin concentration 0.32 ug/L in the activated carbon filter used as an intermediate treatment step to prepare dialysate, and a concentration of 0.4 ug/L was measured in the source water (Hilborn et al., 2013). Out of 44 dialysis patients potentially exposed, 12 were followed up for a period of 8 weeks as they were found to have detectable serum concentrations of microcystins. The median serum concentration in these patients was 0.33 ng/mL. Levels of AST, ALT, gamma-glutamyltransferase (GGT), ALP and bilirubin exceeding their reference ranges were frequently observed throughout the 8 weeks. These were considered consistent with mild to moderate liver injury. In addition, decreased prothrombin time was statistically significantly associated with increased serum microcystin concentration.
During the early part of 1981, the reservoir supplying water to the Armidale region of Australia was affected by a large bloom of Microcystis aeruginosa, a cyanobacteria which produces microcystins, which was treated by the addition of copper sulphate to the water supply. Clinical and plasma enzyme data were collected from all patients treated at the regional hospital for three time periods: a five week period before the first signs of the bloom appeared, the two week period after copper sulphate treatment and a final five week period. The results were also compared between residents of Armidale receiving water from the affected source and those outside the city and in neighbouring towns who had independent water sources.
Analysis of variance showed a statistically significantly higher GGT level during the two-week period of the bloom, only in Armidale residents. ALT also appeared to show an increase in activity in samples from Armidale residents compared with residents with other water sources, but this did not reach statistical significance. The authors concluded that the evidence indicated an increase in liver damage among the population of Armidale during the period of a bloom (Falconer et al., 1993). No exposure data are available from this study.
A WHO review established a provisional tolerable daily intake (TDI) for MC-LR based on the subchronic (90 day) toxicity study in mice by Fawell et al. (1999), in which hepatic lesions were observed at higher doses, supported by the 28-day study in rats by Heinze (1999) (WHO, 2020). An uncertainty factor of 1000 was applied to the no-observed-adverse-effect-level (NOAEL) of 40 µg/kg bodyweight per day in the study in mice. This included uncertainty factors of 10 each for interspecies differences and intraspecies variability in the human population and a further factor of 10 for the use of a subchronic, rather than chronic, study and other limitations in the toxicological data. The resulting provisional TDI is 0.04 µg/kg bodyweight.
The WHO also recommended a provisional short term guidance value for water, based on the same data but applying an uncertainty factor of 100 rather than 1000, omitting the extra uncertainty factor of 10 as the data limitations were considered to primarily affect longer term risks. This is equivalent to a short-term health-based guidance value of 0.4 µg/kg bodyweight. However, it was noted that it was only intended to address risks over very short time periods. This is presumably to ensure that long term intakes remained within the provisional TDI.
The WHO review recommended that exposures to total microcystins, expressed as the equivalent amounts of microcystin-LR, should be compared to the provisional TDI and shorter term guidance value, since while these were based on toxicology data for microcystin-LR, the microcystins occur as mixtures. The WHO review noted the significant uncertainties due to differences in the potencies and toxicokinetics of different microcystins, which can be expected to result in large differences in potency following oral dosing.
Since free microcystins were not detected in fish flesh in any of the samples of eel, roach, pollan, perch or bream, and total (free + protein-bound) microcystins were not detected in any of the samples tested for these of eel, roach, pollan or perch (bream flesh was not tested for total microcystins) it is possible the toxins were not present. However, as discussed above, since some of the microcystins were detected in other parts of the fish it is also possible that they were also present in the fish flesh but at levels below the limits of detection. The limit of detection of the analytical method for total (free + bound) microcystins was 10 µg/kg wet weight.
An upper bound exposure assessment will be conducted. Upper bound exposure assessments assume that substances with analytical results below limits of detection (or other reporting limits) are present at the limit of detection (or reporting limit), in this case the limit of detection of 10 µg/kg. In contrast, lower bound exposure assessments assume that substances with analytical results below limits of detection or reporting limits are entirely absent, i.e. 0 µg/kg. Upper bound exposure assessments may overestimate exposure, whereas lower bound exposure assessments may underestimate exposure.
While edible flesh from the single sample of bream was not tested for total microcystins, free microcystins were not detected and concentrations of total microcystins measured in the viscera of the bream were similar to those for the other species tested. Therefore, it is expected that the results for total microcystins in the flesh of eel, roach, pollan and perch would also apply to the bream.
Eel
Consumption data for eels available through the National Diet and Nutrition Survey (NDNS) were considered for this assessment (Bates et al., 2014, 2016, 2020; Roberts et al., 2018). The NDNS is a programme of surveys designed to assess the diet, nutrient intake and nutritional status of the general population aged 18 months and over living in private households in the UK.
The number of consumers of eel in the NDNS is very small. However, it was considered preferable to use these consumption data rather than consumption data for another species of fish as a proxy. Nevertheless, it introduces some uncertainty in the exposure estimates. In addition, there was a lack of child consumers of eel in the NDNS survey. To address the potential exposures of children who eat eel, an estimate for the consumption of toddlers aged 1-3 years old has been made based on assuming portion sizes of eel are 1/3 those of adults aged 19-64 years (as is typical for other similar foods) and using the mean bodyweight for this age group in the NDNS of 14.6 kg. A similar approach has not been taken for ages 4-18 as their exposures are expected to be between those of toddlers and adults. The consumption data are presented in Table 1 and 2.
Table 1: Chronic consumption of eels (with recipes) in g per person per day. Data from National Diet and Nutrition Survey, years 1-11
Age group | Number of consumers | Mean (g/person/day) | 97.5th Percentile (g/person/day) | Max (g/person/day) |
---|---|---|---|---|
Toddlers (1.5-3yrs)* | 0* | 2.6 | 13 | 16 |
4 - 10 yrs |
0 |
0 | 0 | 0 |
11 - 18 yrs | 0 | 0 | 0 | 0 |
19 - 64 yrs | 3 | 7.9 | 38 | 47 |
65+ yrs | 4 | 54 | 85 | 88 |
Rounded to 2 significant figures.
Consumption or exposure estimates made with a small number of consumers may not be accurate. The number of consumers is less than 60, this should be treated with caution and may not be representative for a large number of consumers.
*Calculated on the assumption toddlers would consume 1/3 of an adult portion and using the average bodyweight (bw) of 14.6 kg.
Table 2: Chronic consumption of eels (with recipes) in g per kg bodyweight (bw) per day. Data from National Diet and Nutrition Survey, years 1-11
Age group | Number of consumers | Mean (g/person/day) | 97.5th Percentile (g/kg bw/day) | Max (g/kg bw/day) |
---|---|---|---|---|
Toddlers (1.5-3yrs)* | 0* | 0.18 | 0.87 | 1.1 |
4 - 10 yrs |
0 |
0 | 0 | 0 |
11 - 18 yrs | 0 | 0 | 0 | 0 |
19 - 64 yrs | 3 | 0.13 | 0.67 | 0.82 |
65+ yrs | 4 | 0.72 | 1 | 1.1 |
Rounded to 2 significant figures.
Consumption or exposure estimates made with a small number of consumers may not be accurate. The number of consumers is less than 60, this should be treated with caution and may not be representative for a large number of consumers.
*Calculated on the assumption toddlers would consume 1/3 of an adult portion and using the average bodyweight (bw) of 14.6 kg.
Using the 97.5th percentile consumption data for each age group in Table 2, and assuming that the total microcystins are present at 10 µg/kg (upper bound approach), the dietary intakes are as shown in Table 3. These are compared with the WHO provisional TDI for microcystins, based on toxicological data for microcystin-LR.
Table 3: Upper bound estimated intakes of total free + bound microcystins in high consumers of eels
Age group | 97.5th Percentile consumption (g/kg bw/day) | Estimated intake at 97.5th percentile (µg/kg bw/day) | Percentage of provisional TDI for microcystins of 0.04 µg/kg bw (to 2 significant figures) |
---|---|---|---|
Toddlers (1.5 - 3 yrs)* | 0.87 | 0.0087 | 22% |
4 - 10 yrs | 0 | 0 | 0% |
11 - 18 yrs | 0 | 0 | 0% |
19 - 64 yrs | 0.67 | 0.0067 | 17% |
65+ yrs | 1 | 0.01 | 25% |
*Calculated on the assumption toddlers would consume 1/3 of an adult portion and using the average bodyweight (bw) of 14.6 kg.
Roach, pollan, perch and bream
There is a lack of consumption data for roach, pollan, perch and bream in the NDNS. Consumption data for trout are used here as a proxy for the consumption of these species of fish individually or in combination (Bates et al., 2014, 2016, 2020; Roberts et al., 2018). The consumption data for trout are presented in Tables 4 and 5.
Table 4: Chronic consumption of trout* (with recipes) in g per person per day. Data from National Diet and Nutrition Survey, years 1-11
Age group | Number of consumers | Mean (g/person/day) | 97.5th Percentile (g/person/day) | Max (g/person/day) |
---|---|---|---|---|
Toddlers (1.5-3yrs) | 7 | 13 | 20 | 20 |
4 - 10 yrs |
6 |
27 | 49 | 50 |
11 - 18 yrs | 3 | 42 | 65 | 73 |
19 - 64 yrs | 42 | 44 | 89 | 110 |
65+ yrs | 29 | 36 | 71 | 89 |
Rounded to 2 significant figures.
Consumption or exposure estimates made with a small number of consumers may not be accurate. The number of consumers is less than 60, this should be treated with caution and may not be representative for a large number of consumers.
*Trout has been used as a proxy for bream, roach, pollan and perch.
Table 5: Chronic consumption of trout* (with recipes) in g per kg bodyweight (bw) per day. Data from National Diet and Nutrition Survey, years 1-11
Age group | Number of consumers | Mean (g/person/day) | 97.5th Percentile (g/kg bw/day) | Max (g/kg bw/day) |
---|---|---|---|---|
Toddlers (1.5-3yrs)* | 7 | 0.81 | 1.4 | 1.4 |
4 - 10 yrs |
6 |
1.3 | 2.8 | 2.9 |
11 - 18 yrs | 3 | 0.62 | 1.1 | 1.3 |
19 - 64 yrs | 42 | 0.58 | 1.5 | 1.5 |
65+ yrs | 29 | 0.49 | 1.1 | 1.4 |
Rounded to 2 significant figures.
Consumption or exposure estimates made with a small number of consumers may not be accurate. The number of consumers is less than 60, this should be treated with caution and may not be representative for a large number of consumers.
*Trout has been used as a proxy for bream, roach, pollan and perch.
Using the 97.5th percentile consumption data for each age group in Table 5, and assuming that the total microcystins are present at 10 µg/kg (upper bound approach), the dietary intakes are as shown in Table 6, below. These are compared with the WHO provisional TDI for microcystins, based on toxicological data for microcystin-LR.
Table 6: Upper bound estimated intakes of total free + bound microcystins in high consumers of roach, pollan, perch and/or bream
Age group | 97.5th Percentile consumption (g/kg bw/day) | Estimated intake at 97.5th percentile (µg/kg bw/day) | Percentage of provisional TDI for microcystins of 0.04 µg/kg bw (to 2 significant figures) |
---|---|---|---|
Toddlers (1.5-3yrs) | 1.4 | 0.014 | 35% |
4 - 10 yrs | 2.8 | 0.028 | 70% |
11 - 18 yrs | 1.1 | 0.011 | 28% |
19 - 64 yrs | 1.5 | 0.015 | 38% |
65+ yrs | 1.1 | 0.011 | 28% |
Consideration of potential dietary exposures if fish were incompletely eviscerated
Since fish may be caught and prepared for consumption not only by food business operators but by recreational anglers, concern has been raised that evisceration may be incomplete or that the edible flesh may become contaminated in the process.
A total of 13 parts of viscera from 9 samples of fish were analysed for total (free + bound) microcystins, with concentrations ranging from 39 µg/kg in a sample of perch gills to 643 µg/kg in a sample of roach intestine. Microcystins were not detected in the flesh. As an exposure scenario, the highest concentration measured, 643 µg/kg in roach intestine will be used and it will be assumed that 10% of the relative proportion of the intestines may be consumed inadvertently with the flesh.
Thus, 100% would mean that edible flesh and intestine would be consumed based on the natural proportions in the fish, while 10% of the relative proportion of intestine to edible flesh is assumed here to represent inadvertent consumption of small amounts of intestine. This is expected to be conservative for long term exposure, in particular when using the highest concentration in any viscera component of all fish samples.
If it is assumed that 12% of the body weight of roach is viscera (general information for fished species in general), and that approximately half of the viscera is intestine, then about 6% of the body weight of the fish would be intestine. In general, the average proportion of a whole fish that is edible flesh is about 58% (MSC, 2021). Thus, the weight of intestines is about 9.4% of the total weight of intestines plus flesh. If it is assumed that 10% of the relative proportion of intestine to flesh may be consumed with the flesh, then fish meat as consumed would be 0.94% intestine with the remaining 99.06% being flesh.
Assuming that the edible flesh contains total microcystins at the limit of detection of 10 µg/kg (upper bound approach), then the total concentration of microcystins in the fish as consumed would be (0.94% x 643 µg/kg) + (99.06% x 10 µg/kg) = 16.0 µg/kg.
Table 7 presents an exposure assessment for roach containing 16.0 µg/kg, using the consumption data for trout from Table 5 as a proxy for roach consumption.
Age group | 97.5th Percentile consumption (g/kg bw/day) | Estimated intake at 97.5th percentile (µg/kg bw/day) | Percentage of provisional TDI for microcystins of 0.04 µg/kg bw (to 2 significant figures) |
---|---|---|---|
Toddlers (1.5 - 3 yrs)* | 1.4 | 0.022 | 55% |
4 - 10 yrs | 2.8 | 0.045 | 110% |
11 - 18 yrs | 1.1 | 0.018 | 45% |
19 - 64 yrs | 1.5 | 0.024 | 60% |
65+ yrs | 1.1 | 0.018 | 45% |
The estimated intakes in Tables 3 and 6 on Exposure Assessment - Microcystins in Fish, are within the provisional TDI in all age groups for high consumers of eel or of roach, pollan, perch and/or bream, even taking the upper bound approach. There are not expected to be other sources of dietary exposure to microcystins. Therefore, no health concern is identified for consuming the flesh of eel, roach, pollan, perch and/or bream.
The estimated intakes in Table 7 on Exposure Assessment - Microcystins in Fish, based on an approximate exposure scenario for the inadvertent consumption of small amounts of viscera together with the edible flesh, marginally exceed the provisional TDI for 4-10 year old high consumers. However, this is only a marginal exceedance in the context of the uncertainty factors used to establish the provisional TDI, which is unlikely to be of toxicological significance. It is not clear that there is truly any exceedance since this exposure assessment used an upper bound approach to the concentration in flesh. Furthermore, the highest concentration in any viscera sample was used for this exposure assessment. Overall, it appears unlikely that consumers will substantially exceed the provisional TDI on a long-term basis due to incomplete evisceration of fish.
To present this risk assessment in a qualitative form, the scales for the frequency of occurrence and severity of foodborne risks and level of associated uncertainty that is described in the multidimensional risk assessment framework outlined by the Advisory Committee on the Microbiological Safety of Food (ACMSF 2020) was used.
This is described in Figure 1.
Figure 1: The probability of an adverse event occurring per serving.
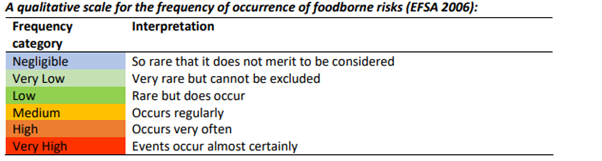
Exposure to microcystins from eating the edible flesh of the tested fish species would not be expected to cause adverse effects in consumers, including if the fish is inadequately eviscerated. Therefore, we consider the frequency of adverse reactions in the general population to be negligible, so rare that it does not merit to be included.
Figure 2: Severity of detriment
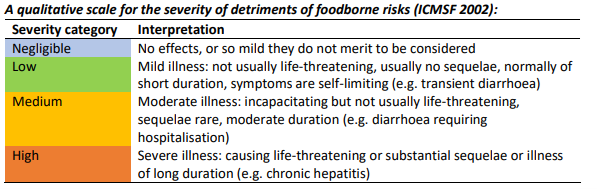
The severity of any adverse effect on the liver would depend on the level of exposure. An outbreak of acute liver injuries occurred in a dialysis clinic in which the dialysis water was contaminated with microcystins and possibly cylindrospermopsin in Brazil in 1996. However, levels of internal exposure to microcystins were very high.
In a second case of dialysis water contaminated with microcystins, increases in biomarkers of hepatic cellular injury and cholestasis exceeding the normal range were frequently observed and were concluded to be consistent with mild-moderate liver injury. Based on the possible very low levels of exposure to microcystins from fish from Lough Neagh, it is considered that any liver injury in consumers of fish, were it to occur, would result from long term exposure and be mild.
Overall, we consider the severity of illness that could potentially occur as a result of exposure to microcystins from consuming edible fish flesh from Lough Neagh to be medium (i.e. moderate illness, incapacitating but not usually life-threatening and of moderate duration).
Figure 3: An assessment of quality of data
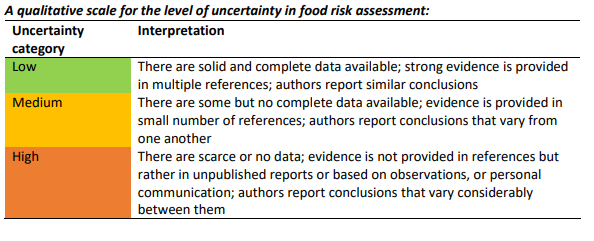
Hepatotoxicity has been reported both in experimental animals (rats and mice) and exposed humans. There are limitations to the toxicological data in experimental animals, which include limited data investigating reproductive toxicity and neurological, immunological and haematological effects, and a lack of chronic toxicity studies in laboratory animals. However, these data limitations were addressed in the provisional TDI used in this risk assessment by the application of an additional uncertainty factor of 10.
While a combined risk assessment is indicated for the microcystins since they are expected to cause the same adverse effects by the same mode of action, there is uncertainty in comparing estimated intakes of total microcystins to the provisional TDI set for MC-LR. There are likely to be significant differences in the toxicological potencies of different microcystins following oral intake due to differences in their inherent potencies and in their toxicokinetics. However, much of the residues detected in viscera were of MC-LR and MC-YR, and the latter has a similar toxic potency following intraperitoneal dosing to MC-LR (WHO, 2020), so the combined approach appears to be reasonable based on the limited data currently available.
Uncertainties in the exposure assessment include the limited consumption data for eels, the use of consumption data for trout as a surrogate for the consumption of for roach, pollan, perch and bream, and the validity of the assumed exposure scenario to address the risks of inadequate evisceration. However, it is exposure averaged over time, rather than on any individual day, which is of relevance to the chronic toxicity of microcystins and the provisional TDI applied in the risk assessment, and considering the consumption data used for adults aged 19-64 years of 89 g/day on a per person basis in the assessment of roach, pollan, perch and/or bream, this is equivalent to 620 g consumption per week, which is equivalent to about 4 portions per week consumption if assuming a portion size of 150 g. This is considered unlikely to underestimate long term consumption of roach, pollan, perch and/or bream by the majority of consumers.
Overall, we consider the level of uncertainty to be medium (i.e. there are some but no complete data available), but that this does not affect the conclusion of the risk assessment that adverse effects are unlikely. The key remaining sources of uncertainty are listed in the next section.
The samples of fish were not taken directly from algal bloom affected areas of the Lough. Therefore, it is not clear whether the Lough contains fish containing higher concentrations of microcystins or additional cyanobacterial toxins to the fish sampled. However, the risks from microcystins relate to long term consumption and it is unlikely that anglers would be catching fish directly within algal blooms.
The risk assessment is based on fish samples taken in autumn 2023 and it is unclear whether microcystin concentrations in fish and in cyanobacteria will change over time.
It is uncertain how much viscera may be consumed inadvertently due to inadequate evisceration or contamination of edible flesh by viscera.
Abramsson-Zetterberg L, Sundh UB, Mattsson R (2010). Cyanobacterial extracts and microcystin-LR are inactive in the micronucleus assay in vivo and in vitro. Mutat Res 699:5-10
Bates B, Lennox A, Prentice A, Bates C, Page P, Nicholson S, Swan G (2014). National Diet and Nutrition Survey Results from Years 1, 2, 3 and 4 (combined) of the Rolling Programme (2008/2009 – 2011/2012).
Bates B, Cox L, Nicholson S, Page P, Prentice A, Steer T, Swan G (2016). National Diet and Nutrition Survey Results from Years 5 and 6 (combined) of the Rolling Programme (2012/2013 – 2013/2014).
Bates B, Collins D, Jones K, Page P, Roberts C, Steer T, Swan G (2020). National Diet and Nutrition Survey Results from years 9, 10 and 11 (combined) of the Rolling Programme (2016/2017 to 2018/2019).
Carmichael WW, Azevedo SMFO, An JS, Molica RJ, Jochimsen EM, Lau S, Rinehart KL, Shaw GR, Eaglesham GK (2001). Human fatalities from cyanobacteria: chemical and biological evidence for cyanotoxins. Environ Health Perspect 109(7):663-8.
DAERA (2023). Summary of blue-green algae monitoring in bathing waters and nearby sites. November 2023. Department of Agriculture, Environment and Rural Affairs. Downloaded 23/2/2024.
Falconer IR, Beresford AM, Runnegar MTC (1983). Evidence of liver damage by toxin from a bloom of the blue-green alga, Microcystis aeruginosa. Med J Aust 1(11):511–14.
Falfushynska H, Kasianchuk N, Siemens E, Henao E, Rzymski P (2023). A review of common cyanotoxins and their effects on fish. Toxics 11(2):118.
Fawell JK, Mitchell RE, Everett DJ, Hill RE (1999). The toxicity of cyanobacterial toxins in the mouse: I. Microcystin–LR. Hum Exper Toxicol 18:162–67.
Heinz R (1999). Toxicity of the cyanobacterial toxin microcystin-LR to rats after 28 days intake with the drinking water. Environ Toxicol 14(1):57-60.
Hilborn ED, Soares RM, Servaites JC, Delgado AG, Magalhães VF, Carmichael WW, Azevedo SMFO (2013). Sublethal microcystin exposure and biochemical outcomes among hemodialysis patients. PLoS One 8(7):1–9.
IARC (International Agency for Research on Cancer) (2010). Ingested nitrate and nitrite, and cyanobacterial peptide toxins. Geneva: IARC (IARC Monographs on the Evaluation of Carcinogenic Risks to Humans, Vol. 94).
Mohamed ZA, Bakr A, Soliman HA (2018). Bioavailability of bound microcystins in mice orally fed with contaminated tilapia edible tissues: Implications to human health. Toxicon 151:34-36.
MSC (2021). MSC Insights: Sustainable fishing, higher yields and the global food supply. Marine Stewardship Council, January 2021. Downloaded 7/12/2023.
Roberts C, Steer T, Maplethorpe N, Cox L, Meadows S, Page P, Nicholson S, Swan G (2018). National Diet and Nutrition Survey Results from Years 7 and 8 (combined) of the Rolling Programme (2014/2015 – 2015/2016).
Testai E, Buratti FM, Funari E, Manganelli M, Vichi S, Arnich N, Biré R, Fessard V, Sialehaamoa A (2016). Review and analysis of occurrence, exposure and toxicity of cyanobacteria toxins in food. EFSA supporting publication 2016:EN-998. 309 pp. Downloaded on 18/9/2023.
Ueno Y, Makita Y, Nagata S Tsutsumi T, Yoshada F, Tamura S-I, Sekijima M, Tashiro F, Harada T, Yoshida T (1999). No chronic oral toxicity of a low-dose of microcystin-LR, a cyanobacterial hepatoxin, in female Balb/C mice. Environ Toxicol 14(1):45-55.
WHO (2020). Cyanobacterial toxins: microcystins. Background document for development of WHO Guidelines for drinking-water quality and Guidelines for safe recreational water environments. Geneva, World Health Organization (WHO/HEP/ECH/WSH/2020.6). Downloaded on 18/9/2023.